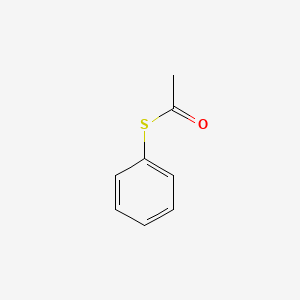
S-Phenyl thioacetate
Overview
Description
S-Phenyl thioacetate (C₈H₈OS, CAS 934-87-2), also known as phenyl thioacetate or S-phenyl ethanethioate, is a sulfur-containing ester with a phenyl group bonded to a thioacetate moiety. Its molecular structure enables versatile reactivity in organic synthesis, particularly as an acetyl donor in acetylation reactions . Key applications include:
- Catalytic acetylation: Used in DMAP-catalyzed lysine acetylation studies to probe transition-state mechanisms .
- Enzymatic substrates: Serves as a substrate for paraoxonase-1 (PON1) to study arylesterase activity and carbamate inhibition .
- Synthetic intermediates: Employed in TiCl₄-promoted aldol condensations to generate unsaturated selenoesters and thioamides .
Preparation Methods
Synthetic Routes and Reaction Conditions:
Thioesterification: One common method to synthesize S-Phenyl thioacetate involves the reaction of thiophenol with acetic anhydride or acetyl chloride in the presence of a base such as pyridine.
Nucleophilic Substitution: Another method involves the nucleophilic substitution of a halide (e.g., acetyl chloride) with thiophenol in the presence of a base like potassium carbonate.
Industrial Production Methods: Industrial production of this compound often employs similar synthetic routes but on a larger scale. The use of continuous flow reactors and optimized reaction conditions ensures high yield and purity of the product. The reactions are typically carried out under controlled temperature and pressure to maximize efficiency .
Chemical Reactions Analysis
Types of Reactions:
Oxidation: S-Phenyl thioacetate can undergo oxidation reactions to form sulfoxides and sulfones.
Reduction: Reduction of this compound can yield thiophenol and acetic acid.
Substitution: this compound can participate in nucleophilic substitution reactions, where the acetyl group is replaced by other nucleophiles.
Common Reagents and Conditions:
Oxidation: Hydrogen peroxide, m-chloroperbenzoic acid.
Reduction: Lithium aluminum hydride.
Substitution: Bromine, chlorine, potassium carbonate.
Major Products Formed:
Oxidation: Sulfoxides, sulfones.
Reduction: Thiophenol, acetic acid.
Substitution: Acetyl halides, phenyl disulfide.
Scientific Research Applications
Chemical Synthesis
1.1 Acetylation Reactions
S-Phenyl thioacetate serves as an acetyl donor in various acetylation reactions, particularly in the modification of nucleosomal histones. A study demonstrated that this compound can be utilized alongside DMAP (4-Dimethylaminopyridine) to achieve high-yield acetylation of lysine residues on histones, which is crucial for epigenetic research . The mechanism involves the formation of a six-membered transition state that stabilizes the reaction, enhancing the acetylation efficiency.
1.2 Thiol–Thioester Exchange
The kinetics of thiol–thioester exchange involving this compound have been extensively studied. Research indicates that this compound exhibits a relatively long half-life for hydrolysis in neutral water conditions, making it suitable for applications in self-assembly and reversible binding systems . The exchange rates can be optimized for various conditions, which is beneficial for developing new materials and biochemical systems.
Biochemical Studies
2.1 Mechanistic Studies
This compound has been used to probe the mechanisms of aminolysis reactions. It acts as a substrate to investigate how the thiocarbonyl group influences reaction pathways and kinetics. Studies have shown that the stability of intermediates formed during these reactions can greatly affect the overall reaction rates, providing insights into reaction mechanisms involving thioesters .
2.2 Enzyme Activity Modulation
Research has also explored how this compound can influence enzyme activity. For instance, its interactions with paraoxonase 1 (PON1) have been studied to understand how carbamates affect this enzyme's activity. Such investigations are vital for understanding detoxification processes in biological systems .
Therapeutic Potential
3.1 Drug Development
The properties of this compound suggest potential applications in drug development, particularly in designing inhibitors or modulators for specific biochemical pathways. Its ability to participate in selective acetylation reactions opens avenues for creating compounds that can target specific proteins or enzymes involved in disease processes.
3.2 Epigenetic Modulation
Given its role in histone acetylation, this compound may have implications in epigenetic therapies aimed at treating diseases linked to aberrant histone modifications, such as cancer. The ability to manipulate histone acetylation levels could provide a novel approach to regulating gene expression and cellular behavior .
Summary of Findings
The applications of this compound span across various scientific disciplines, highlighting its versatility as a chemical reagent and its potential impact on biological research and therapeutic development.
Application Area | Details |
---|---|
Chemical Synthesis | Used as an acetyl donor; enhances lysine acetylation efficiency; involved in thiol–thioester exchange |
Biochemical Studies | Investigates reaction mechanisms; modulates enzyme activity; studies interactions with PON1 |
Therapeutic Potential | Potential use in drug development; implications for epigenetic therapies |
Mechanism of Action
The mechanism of action of S-Phenyl thioacetate primarily involves its reactivity as a thioester. In enzymatic reactions, it acts as a substrate for esterases, which catalyze the hydrolysis of the thioester bond, releasing thiophenol and acetic acid. This reaction is crucial for studying enzyme kinetics and understanding the catalytic mechanisms of esterases .
Comparison with Similar Compounds
Reactivity in Acetylation Reactions
S-Phenyl thioacetate (PhSAc) is compared to structurally related acetyl donors, such as phenyl cyanoacetate (Ph(CN)OAc), to evaluate the influence of leaving-group basicity on reaction efficiency.
Key Insight : The reduced basicity of PhSAc’s leaving group limits its ability to form a six-membered transition state in DMAP-catalyzed reactions, resulting in slower acetylation compared to Ph(CN)OAc .
Comparison with Methyl Thioacetate :
- Methyl thioacetate is highly unstable and hydrolyzes rapidly to acetic acid, limiting its utility in synthetic applications .
- This compound exhibits superior stability under acidic and basic conditions, enabling its use in high-temperature catalytic systems (>200°C) .
Enzymatic Interactions and Inhibition
Paraoxonase-1 (PON1) hydrolyzes PhSAc, but its activity is inhibited by carbamates. Inhibition constants (Kᵢ) vary:
Carbamate | Kᵢ (mM) for PON1 Inhibition | Relative Affinity vs. PhSAc |
---|---|---|
Ro 02-0683 | 0.9 | ~3–40× lower |
Physostigmine | 1.1 | ~3–40× lower |
Carbofuran | 0.4 | ~10× lower |
Bambuterol | 2.2 | ~2× lower |
Key Insight: Carbamates exhibit competitive inhibition via non-covalent interactions with PON1’s active site, reducing arylesterase activity toward PhSAc .
Biological Activity
S-Phenyl thioacetate (CAS 934-87-2) is a sulfur-containing organic compound that has garnered attention in various fields of biological research due to its potential pharmacological properties. This article explores the biological activity of this compound, including its antibacterial, antifungal, and enzymatic effects, supported by data tables and case studies.
Chemical Structure and Properties
This compound is characterized by the molecular formula and a molecular weight of approximately 152.21 g/mol. The compound features an acetylthio group attached to a phenyl ring, which plays a crucial role in its biological interactions.
Antimicrobial Activity
Research indicates that this compound exhibits significant antimicrobial activity against various bacterial and fungal strains. A study reported the synthesis of several thiazole derivatives, which included this compound as a precursor. The synthesized compounds were evaluated for their antibacterial activity against Escherichia coli and Staphylococcus aureus, as well as antifungal activity against Aspergillus niger and Candida albicans.
Table 1: Antimicrobial Activity of this compound Derivatives
Compound | Bacterial Strain | Zone of Inhibition (mm) | Antifungal Activity |
---|---|---|---|
This compound | E. coli | 15 | Moderate |
S. aureus | 18 | Moderate | |
A. niger | - | Weak | |
C. albicans | - | Weak |
The results indicate that while this compound shows moderate antibacterial activity, its antifungal effects are less pronounced .
Enzymatic Activity
This compound has also been studied for its role as a substrate in enzymatic reactions. Specifically, it has been used to measure esterase activity in various biological systems. Paraoxonase-1 (PON1), an enzyme associated with high-density lipoprotein (HDL), hydrolyzes a broad spectrum of esters, including this compound. This interaction suggests potential implications for drug metabolism and detoxification processes in mammals .
Case Study: Esterase Activity Measurement
In a study assessing the influence of carbamates on PON1 activity, this compound was employed as a substrate to evaluate the enzyme's efficiency in hydrolyzing different esters. The findings highlighted that variations in substrate structure could significantly affect enzymatic activity, thereby influencing pharmacological outcomes .
Toxicological Considerations
While the biological activities of this compound are promising, it is essential to consider its toxicological profile. Initial assessments indicate that while it exhibits antimicrobial properties, further studies are required to evaluate its safety and potential side effects in vivo.
Q & A
Basic Research Questions
Q. What are the standard synthetic routes for preparing S-phenyl thioacetate in laboratory settings?
this compound is typically synthesized via nucleophilic substitution or esterification reactions. For instance, thioacetate displacement reactions using potassium thioacetate (KSAc) under heated conditions in polar aprotic solvents like DMF have been employed to introduce the thioacetate moiety . Alternatively, direct thioacetylation of thiophenol derivatives with acetyl chloride or acetic anhydride in the presence of a base (e.g., pyridine) is a common method. Reaction progress is monitored via TLC, and purification is achieved through column chromatography or recrystallization.
Q. Which spectroscopic techniques are employed to characterize this compound, and what key spectral features confirm its structure?
Key characterization methods include:
- Infrared (IR) Spectroscopy : The C=O stretch of the thioacetate group appears at ~1680–1700 cm⁻¹, while the C-S bond vibration is observed near 650–700 cm⁻¹ .
- Raman Spectroscopy : Complementing IR, Raman peaks at ~1100 cm⁻¹ (C-S stretching) and ~500 cm⁻¹ (S-C=O bending) confirm the thioester linkage .
- ¹H NMR : Signals at δ ~2.4 ppm (singlet for acetyl CH₃) and δ ~7.3–7.5 ppm (aromatic protons) are diagnostic .
Q. What are the primary applications of this compound in basic organic synthesis?
It serves as a versatile intermediate in:
- Thiol Protection/Deprotection : The acetyl group acts as a protecting group for thiols, which can be cleaved using hydrazine or other nucleophiles .
- Asymmetric Synthesis : As a thioester donor in Mannich reactions to form β-amino carbonyl compounds .
Advanced Research Questions
Q. How can computational methods like DFT elucidate the vibrational and electronic properties of this compound?
Density Functional Theory (DFT) calculations at the 6-311++G(d,p) basis set level predict molecular geometry, vibrational frequencies, and electron distribution. For example, DFT simulations align with experimental IR/Raman data, confirming the planarity of the thioester group and delocalization of π-electrons in the C=S bond . These studies aid in rationalizing reactivity patterns, such as nucleophilic attack at the carbonyl carbon.
Q. What methodological considerations are critical when using this compound in asymmetric Mannich reactions?
Key parameters include:
- Enolate Geometry : Silyl enolates (e.g., from this compound) must adopt the E-configuration to ensure stereoselective addition to imines .
- Catalyst Choice : Chiral Lewis acids (e.g., BINOL-derived catalysts) enhance enantioselectivity.
- Solvent and Temperature : Reactions in anhydrous DMF at –20°C to 0°C minimize side reactions .
Q. How does the nonspecificity of this compound in enzymatic assays impact experimental design?
In hepatic carboxylesterase (CbE) assays, this compound’s nonspecific hydrolysis by multiple esterase isoforms complicates data interpretation. To mitigate this:
- Substrate Alternatives : Use p-nitrophenyl acetate (pNPA) or α-naphthyl acetate (α-NA) for higher specificity .
- Inhibitor Controls : Include eserine (a cholinesterase inhibitor) to isolate CbE activity .
Q. How does this compound contribute to nanoparticle-based detection systems, and what experimental parameters optimize sensitivity?
In paraoxonase (PON1) activity assays, this compound hydrolysis generates thiophenol, which quenches CdS quantum dot (QD) fluorescence by surface binding or Cd²⁺ complexation . Optimization requires:
- pH Control : Maintain pH ~8 to balance QD stability and enzymatic activity.
- Stabilizers : Add sodium thiophosphate (Na₃PO₃S) to stabilize QDs and reduce false negatives .
- Kinetic Monitoring : Track fluorescence decay rates (λₑₓ = 350 nm, λₑₘ = 520 nm) for real-time enzyme quantification.
Q. What safety protocols are essential for handling this compound in research laboratories?
- Personal Protective Equipment (PPE) : Use nitrile gloves, lab coats, and safety goggles. Work in a fume hood to avoid inhalation .
- Spill Management : Neutralize spills with sodium bicarbonate, then adsorb with inert material (e.g., vermiculite) .
- Disposal : Comply with local regulations (e.g., US EPA 40 CFR Part 261) for hazardous waste disposal. Incinerate in a licensed facility .
Q. Data Contradiction Analysis
Q. How should researchers resolve contradictions in substrate specificity across enzymatic studies using this compound?
Discrepancies arise from interspecies enzyme variability (e.g., Senegalese sole vs. mammalian CbE). To address this:
- Species-Specific Validation : Pre-test substrate specificity using purified isoforms.
- Cross-Validation : Compare results with orthogonal assays (e.g., HPLC-based hydrolysis quantification) .
Q. What factors influence the stability of this compound during long-term storage?
Degradation via hydrolysis or oxidation is accelerated by moisture, light, and elevated temperatures. Stability can be enhanced by:
- Storage Conditions : Keep in anhydrous, amber vials under argon at –20°C .
- Purity Checks : Regularly analyze via HPLC to detect degradation products (e.g., thiophenol or acetic acid) .
Properties
IUPAC Name |
S-phenyl ethanethioate | |
---|---|---|
Source | PubChem | |
URL | https://pubchem.ncbi.nlm.nih.gov | |
Description | Data deposited in or computed by PubChem | |
InChI |
InChI=1S/C8H8OS/c1-7(9)10-8-5-3-2-4-6-8/h2-6H,1H3 | |
Source | PubChem | |
URL | https://pubchem.ncbi.nlm.nih.gov | |
Description | Data deposited in or computed by PubChem | |
InChI Key |
WBISVCLTLBMTDS-UHFFFAOYSA-N | |
Source | PubChem | |
URL | https://pubchem.ncbi.nlm.nih.gov | |
Description | Data deposited in or computed by PubChem | |
Canonical SMILES |
CC(=O)SC1=CC=CC=C1 | |
Source | PubChem | |
URL | https://pubchem.ncbi.nlm.nih.gov | |
Description | Data deposited in or computed by PubChem | |
Molecular Formula |
C8H8OS | |
Source | PubChem | |
URL | https://pubchem.ncbi.nlm.nih.gov | |
Description | Data deposited in or computed by PubChem | |
DSSTOX Substance ID |
DTXSID00239419 | |
Record name | Thiophenyl acetate | |
Source | EPA DSSTox | |
URL | https://comptox.epa.gov/dashboard/DTXSID00239419 | |
Description | DSSTox provides a high quality public chemistry resource for supporting improved predictive toxicology. | |
Molecular Weight |
152.22 g/mol | |
Source | PubChem | |
URL | https://pubchem.ncbi.nlm.nih.gov | |
Description | Data deposited in or computed by PubChem | |
CAS No. |
934-87-2 | |
Record name | Ethanethioic acid, S-phenyl ester | |
Source | CAS Common Chemistry | |
URL | https://commonchemistry.cas.org/detail?cas_rn=934-87-2 | |
Description | CAS Common Chemistry is an open community resource for accessing chemical information. Nearly 500,000 chemical substances from CAS REGISTRY cover areas of community interest, including common and frequently regulated chemicals, and those relevant to high school and undergraduate chemistry classes. This chemical information, curated by our expert scientists, is provided in alignment with our mission as a division of the American Chemical Society. | |
Explanation | The data from CAS Common Chemistry is provided under a CC-BY-NC 4.0 license, unless otherwise stated. | |
Record name | Thiophenyl acetate | |
Source | ChemIDplus | |
URL | https://pubchem.ncbi.nlm.nih.gov/substance/?source=chemidplus&sourceid=0000934872 | |
Description | ChemIDplus is a free, web search system that provides access to the structure and nomenclature authority files used for the identification of chemical substances cited in National Library of Medicine (NLM) databases, including the TOXNET system. | |
Record name | Thiophenyl acetate | |
Source | EPA DSSTox | |
URL | https://comptox.epa.gov/dashboard/DTXSID00239419 | |
Description | DSSTox provides a high quality public chemistry resource for supporting improved predictive toxicology. | |
Record name | S-phenyl thioacetate | |
Source | European Chemicals Agency (ECHA) | |
URL | https://echa.europa.eu/substance-information/-/substanceinfo/100.012.086 | |
Description | The European Chemicals Agency (ECHA) is an agency of the European Union which is the driving force among regulatory authorities in implementing the EU's groundbreaking chemicals legislation for the benefit of human health and the environment as well as for innovation and competitiveness. | |
Explanation | Use of the information, documents and data from the ECHA website is subject to the terms and conditions of this Legal Notice, and subject to other binding limitations provided for under applicable law, the information, documents and data made available on the ECHA website may be reproduced, distributed and/or used, totally or in part, for non-commercial purposes provided that ECHA is acknowledged as the source: "Source: European Chemicals Agency, http://echa.europa.eu/". Such acknowledgement must be included in each copy of the material. ECHA permits and encourages organisations and individuals to create links to the ECHA website under the following cumulative conditions: Links can only be made to webpages that provide a link to the Legal Notice page. | |
Record name | S-PHENYL THIOACETATE | |
Source | FDA Global Substance Registration System (GSRS) | |
URL | https://gsrs.ncats.nih.gov/ginas/app/beta/substances/L1K5I18NJ8 | |
Description | The FDA Global Substance Registration System (GSRS) enables the efficient and accurate exchange of information on what substances are in regulated products. Instead of relying on names, which vary across regulatory domains, countries, and regions, the GSRS knowledge base makes it possible for substances to be defined by standardized, scientific descriptions. | |
Explanation | Unless otherwise noted, the contents of the FDA website (www.fda.gov), both text and graphics, are not copyrighted. They are in the public domain and may be republished, reprinted and otherwise used freely by anyone without the need to obtain permission from FDA. Credit to the U.S. Food and Drug Administration as the source is appreciated but not required. | |
Retrosynthesis Analysis
AI-Powered Synthesis Planning: Our tool employs the Template_relevance Pistachio, Template_relevance Bkms_metabolic, Template_relevance Pistachio_ringbreaker, Template_relevance Reaxys, Template_relevance Reaxys_biocatalysis model, leveraging a vast database of chemical reactions to predict feasible synthetic routes.
One-Step Synthesis Focus: Specifically designed for one-step synthesis, it provides concise and direct routes for your target compounds, streamlining the synthesis process.
Accurate Predictions: Utilizing the extensive PISTACHIO, BKMS_METABOLIC, PISTACHIO_RINGBREAKER, REAXYS, REAXYS_BIOCATALYSIS database, our tool offers high-accuracy predictions, reflecting the latest in chemical research and data.
Strategy Settings
Precursor scoring | Relevance Heuristic |
---|---|
Min. plausibility | 0.01 |
Model | Template_relevance |
Template Set | Pistachio/Bkms_metabolic/Pistachio_ringbreaker/Reaxys/Reaxys_biocatalysis |
Top-N result to add to graph | 6 |
Feasible Synthetic Routes
Disclaimer and Information on In-Vitro Research Products
Please be aware that all articles and product information presented on BenchChem are intended solely for informational purposes. The products available for purchase on BenchChem are specifically designed for in-vitro studies, which are conducted outside of living organisms. In-vitro studies, derived from the Latin term "in glass," involve experiments performed in controlled laboratory settings using cells or tissues. It is important to note that these products are not categorized as medicines or drugs, and they have not received approval from the FDA for the prevention, treatment, or cure of any medical condition, ailment, or disease. We must emphasize that any form of bodily introduction of these products into humans or animals is strictly prohibited by law. It is essential to adhere to these guidelines to ensure compliance with legal and ethical standards in research and experimentation.