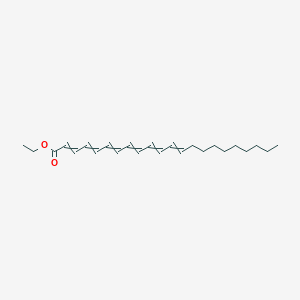
Ethyl docosahexaenate
Overview
Description
Ethyl docosahexaenate, also known as ethyl (4Z,7Z,10Z,13Z,16Z,19Z)-docosahexaenoate, is a long-chain fatty acid ethyl ester derived from docosahexaenoic acid. It is a polyunsaturated omega-3 fatty acid that plays a crucial role in various biological processes. This compound is commonly found in fish oils and is known for its significant health benefits, particularly in relation to brain function and cardiovascular health .
Mechanism of Action
Target of Action
Ethyl docosahexaenoate, also known as Ethyl cis-4,7,10,13,16,19-Docosahexaenoate, is a long-chain fatty acid ethyl ester . It is derived from the formal condensation of the carboxy group of docosahexaenoic acid (DHA) with the hydroxy group of ethanol . The primary targets of Ethyl docosahexaenoate are the apolipoprotein B-containing lipoproteins .
Mode of Action
Ethyl docosahexaenoate interacts with its targets, the apolipoprotein B-containing lipoproteins, and affects their function .
Biochemical Pathways
The biochemical pathways affected by Ethyl docosahexaenoate are those involving the metabolism of apolipoprotein B-containing lipoproteins . The compound reduces the production of very-low-density lipoprotein (VLDL) in the liver, likely through increased hepatic fatty acid oxidation . This leads to decreased fasting and postprandial serum triglyceride levels .
Pharmacokinetics
It is known that dha, from which ethyl docosahexaenoate is derived, is rapidly absorbed from the gastrointestinal tract and distributed throughout the body .
Result of Action
The action of Ethyl docosahexaenoate results in decreased serum triglyceride levels, both fasting and postprandial . This is achieved through reduced production of VLDL in the liver and increased clearance of chylomicrons .
Action Environment
The action, efficacy, and stability of Ethyl docosahexaenoate can be influenced by various environmental factors. For instance, the compound’s oxidative stability can be affected by the presence of oxygen
Biochemical Analysis
Biochemical Properties
Ethyl docosahexaenate is involved in several biochemical reactions, primarily due to its structural similarity to DHA. It interacts with various enzymes, proteins, and other biomolecules. One of the key enzymes it interacts with is phospholipase A2, which hydrolyzes the ester bond, releasing DHA. This interaction is crucial for the incorporation of DHA into cell membranes, where it influences membrane fluidity and function. Additionally, this compound interacts with cyclooxygenase and lipoxygenase enzymes, leading to the production of bioactive lipid mediators that play roles in inflammation and cell signaling .
Cellular Effects
This compound exerts several effects on different cell types and cellular processes. In neuronal cells, it enhances membrane fluidity, which is essential for proper synaptic function and neurotransmission. It also influences cell signaling pathways, such as the PI3K/Akt pathway, promoting cell survival and neuroprotection. In immune cells, this compound modulates the expression of inflammatory cytokines, thereby exerting anti-inflammatory effects. Furthermore, it affects gene expression by acting as a ligand for nuclear receptors, such as PPARs, which regulate genes involved in lipid metabolism and inflammation .
Molecular Mechanism
At the molecular level, this compound exerts its effects through several mechanisms. It binds to and activates PPARs, leading to changes in gene expression that promote anti-inflammatory and lipid-regulating effects. Additionally, it can be incorporated into cell membranes, where it influences membrane-associated proteins and receptors. This compound also inhibits the activity of enzymes like cyclooxygenase, reducing the production of pro-inflammatory eicosanoids. These molecular interactions contribute to its overall beneficial effects on cellular function and health .
Temporal Effects in Laboratory Settings
In laboratory settings, the effects of this compound can vary over time. It is relatively stable under physiological conditions but can undergo oxidation, leading to the formation of lipid peroxides. Long-term studies have shown that chronic administration of this compound can lead to sustained incorporation into cell membranes, resulting in prolonged neuroprotective and anti-inflammatory effects. Its stability and efficacy can be influenced by factors such as storage conditions and exposure to light and oxygen .
Dosage Effects in Animal Models
The effects of this compound in animal models are dose-dependent. At low to moderate doses, it has been shown to improve cognitive function and reduce inflammation in models of neurodegenerative diseases. At high doses, it can lead to adverse effects, such as oxidative stress and lipid peroxidation. These threshold effects highlight the importance of optimizing dosage to achieve therapeutic benefits while minimizing potential toxicity .
Metabolic Pathways
This compound is metabolized through pathways similar to those of DHA. It undergoes beta-oxidation in the mitochondria, leading to the production of acetyl-CoA, which enters the citric acid cycle for energy production. Additionally, it can be converted back to DHA through hydrolysis by esterases. This metabolic flexibility allows this compound to contribute to various physiological processes, including energy metabolism and the synthesis of bioactive lipid mediators .
Transport and Distribution
Within cells and tissues, this compound is transported and distributed by binding to lipid transport proteins, such as albumin and lipoproteins. It is incorporated into cell membranes, where it can influence membrane fluidity and function. The distribution of this compound is uneven, with higher concentrations found in tissues with high metabolic activity, such as the brain and liver. This selective distribution is crucial for its role in supporting cellular function and health .
Subcellular Localization
This compound is primarily localized in the phospholipid bilayer of cell membranes. It can also be found in intracellular organelles, such as mitochondria and the endoplasmic reticulum, where it influences membrane dynamics and function. The subcellular localization of this compound is directed by its incorporation into phospholipids, which are targeted to specific cellular compartments. This localization is essential for its role in modulating cellular processes and maintaining cellular homeostasis .
Preparation Methods
Ethyl docosahexaenate can be synthesized through the selective alcoholysis of fatty acid ethyl esters. One common method involves the use of immobilized lipase enzymes, such as Rhizomucor miehei lipase, to catalyze the reaction between ethyl esters and lauryl alcohol. This process efficiently enriches this compound from sources like tuna oil. The reaction is typically carried out at 30°C with shaking, and the optimal conditions include a mixture of ethyl esters and lauryl alcohol in a 1:3 molar ratio, with 4 wt% of the immobilized lipase .
Chemical Reactions Analysis
Ethyl docosahexaenate undergoes various chemical reactions, including oxidation and autoxidation. The autoxidation kinetics of this compound have been studied extensively, revealing that it is highly susceptible to oxidative degradation due to its multiple double bonds. Common reagents used in these reactions include oxygen and various antioxidants to prevent or slow down the oxidation process. The major products formed from these reactions are typically oxidized derivatives of the original compound .
Scientific Research Applications
Ethyl docosahexaenate has a wide range of scientific research applications. In chemistry, it is used as a model compound to study the oxidation kinetics of polyunsaturated fatty acids. In biology and medicine, it is investigated for its potential therapeutic effects on brain health, cardiovascular health, and inflammatory conditions. It is also used in the food industry as a dietary supplement to enhance the nutritional value of various products .
Comparison with Similar Compounds
Ethyl docosahexaenate is often compared with other omega-3 fatty acid ethyl esters, such as ethyl eicosapentaenoate. While both compounds share similar health benefits, this compound is unique in its higher degree of unsaturation, which makes it more susceptible to oxidation but also more effective in modulating membrane fluidity and function. Other similar compounds include ethyl eicosapentaenoate and ethyl linoleate, which differ in their chain length and degree of unsaturation .
Properties
IUPAC Name |
ethyl docosa-2,4,6,8,10,12-hexaenoate | |
---|---|---|
Source | PubChem | |
URL | https://pubchem.ncbi.nlm.nih.gov | |
Description | Data deposited in or computed by PubChem | |
InChI |
InChI=1S/C24H36O2/c1-3-5-6-7-8-9-10-11-12-13-14-15-16-17-18-19-20-21-22-23-24(25)26-4-2/h12-23H,3-11H2,1-2H3 | |
Source | PubChem | |
URL | https://pubchem.ncbi.nlm.nih.gov | |
Description | Data deposited in or computed by PubChem | |
InChI Key |
TYLNXKAVUJJPMU-UHFFFAOYSA-N | |
Source | PubChem | |
URL | https://pubchem.ncbi.nlm.nih.gov | |
Description | Data deposited in or computed by PubChem | |
Canonical SMILES |
CCCCCCCCCC=CC=CC=CC=CC=CC=CC(=O)OCC | |
Source | PubChem | |
URL | https://pubchem.ncbi.nlm.nih.gov | |
Description | Data deposited in or computed by PubChem | |
Molecular Formula |
C24H36O2 | |
Source | PubChem | |
URL | https://pubchem.ncbi.nlm.nih.gov | |
Description | Data deposited in or computed by PubChem | |
DSSTOX Substance ID |
DTXSID60276426 | |
Record name | Ethyl 2,4,6,8,10,12-docosahexaenoate | |
Source | EPA DSSTox | |
URL | https://comptox.epa.gov/dashboard/DTXSID60276426 | |
Description | DSSTox provides a high quality public chemistry resource for supporting improved predictive toxicology. | |
Molecular Weight |
356.5 g/mol | |
Source | PubChem | |
URL | https://pubchem.ncbi.nlm.nih.gov | |
Description | Data deposited in or computed by PubChem | |
CAS No. |
1020718-25-5 | |
Record name | Ethyl 2,4,6,8,10,12-docosahexaenoate | |
Source | EPA DSSTox | |
URL | https://comptox.epa.gov/dashboard/DTXSID60276426 | |
Description | DSSTox provides a high quality public chemistry resource for supporting improved predictive toxicology. | |
Retrosynthesis Analysis
AI-Powered Synthesis Planning: Our tool employs the Template_relevance Pistachio, Template_relevance Bkms_metabolic, Template_relevance Pistachio_ringbreaker, Template_relevance Reaxys, Template_relevance Reaxys_biocatalysis model, leveraging a vast database of chemical reactions to predict feasible synthetic routes.
One-Step Synthesis Focus: Specifically designed for one-step synthesis, it provides concise and direct routes for your target compounds, streamlining the synthesis process.
Accurate Predictions: Utilizing the extensive PISTACHIO, BKMS_METABOLIC, PISTACHIO_RINGBREAKER, REAXYS, REAXYS_BIOCATALYSIS database, our tool offers high-accuracy predictions, reflecting the latest in chemical research and data.
Strategy Settings
Precursor scoring | Relevance Heuristic |
---|---|
Min. plausibility | 0.01 |
Model | Template_relevance |
Template Set | Pistachio/Bkms_metabolic/Pistachio_ringbreaker/Reaxys/Reaxys_biocatalysis |
Top-N result to add to graph | 6 |
Feasible Synthetic Routes
Disclaimer and Information on In-Vitro Research Products
Please be aware that all articles and product information presented on BenchChem are intended solely for informational purposes. The products available for purchase on BenchChem are specifically designed for in-vitro studies, which are conducted outside of living organisms. In-vitro studies, derived from the Latin term "in glass," involve experiments performed in controlled laboratory settings using cells or tissues. It is important to note that these products are not categorized as medicines or drugs, and they have not received approval from the FDA for the prevention, treatment, or cure of any medical condition, ailment, or disease. We must emphasize that any form of bodily introduction of these products into humans or animals is strictly prohibited by law. It is essential to adhere to these guidelines to ensure compliance with legal and ethical standards in research and experimentation.