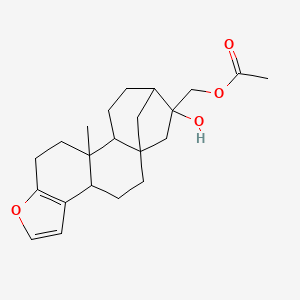
Cafestol acetate
Overview
Description
Cafestol acetate is a natural diterpene compound derived from coffee beans. It is an esterified form of cafestol, which is known for its various biological activities. This compound has garnered attention due to its potential pharmacological properties, including anti-inflammatory, anti-carcinogenic, and hepatoprotective effects .
Mechanism of Action
Target of Action
Cafestol acetate primarily targets several key proteins and receptors in the body. It acts as an agonist ligand for the nuclear receptors farnesoid X receptor and pregnane X receptor . These receptors play a crucial role in regulating lipid metabolism, inflammation, and endothelial function, which are pivotal in cardiovascular pathophysiology .
Mode of Action
This compound interacts with its targets by blocking cholesterol homeostasis . It suppresses enzymes involved in bile acid synthesis, such as sterol 27-hydroxylase and cholesterol 7 alpha-hydroxylase . This leads to a decrease in major enzymes’ mRNA levels . Additionally, it has been shown to induce apoptosis and inhibit cell growth in various cancer models .
Biochemical Pathways
This compound affects multiple biochemical pathways. It influences lipid metabolism through modulation of fat oxidation, bile acid synthesis, VLDL production, and lipoprotein concentrations . It also down-regulates inflammation mediators, increases glutathione (GSH), induces apoptosis of tumor cells, and inhibits angiogenesis .
Pharmacokinetics
About 70% of the consumption of this compound can be absorbed in the small intestine . In theory, glucuronidation and sulphation are the major pathways of xenobiotic biotransformation in mammalian species, which occurs largely in the liver, to produce water-soluble products that can be excreted by urine .
Result of Action
The molecular and cellular effects of this compound’s action are diverse. It can increase serum cholesterol levels , but it may also have beneficial effects, such as decreasing serum lipoprotein (a) concentrations and attenuating fibrosis . In cancer cells, it exhibits pro-apoptotic, cytotoxic, anti-proliferative, and anti-migratory properties .
Action Environment
The action, efficacy, and stability of this compound can be influenced by various environmental factors. For instance, the type of coffee brew can affect the amount of this compound ingested, as it is present in higher amounts in unfiltered coffee like Turkish coffee . Furthermore, the impact of this compound on health can vary depending on individual dietary habits and genetic factors .
Biochemical Analysis
Biochemical Properties
Cafestol acetate plays a crucial role in various biochemical reactions. It interacts with several enzymes, proteins, and other biomolecules, influencing their activity and function. One of the primary interactions of this compound is with the nuclear receptors farnesoid X receptor (FXR) and pregnane X receptor (PXR). This compound acts as an agonist ligand for these receptors, modulating cholesterol homeostasis and lipid metabolism . Additionally, this compound has been shown to increase the levels of glutathione (GSH) by stimulating γ-glutamylcysteine synthetase, which plays a vital role in antioxidant defense .
Cellular Effects
This compound exerts significant effects on various types of cells and cellular processes. It has been demonstrated to induce apoptosis in colorectal and renal cancer cells, highlighting its potential as an anti-carcinogenic agent . Furthermore, this compound influences cell signaling pathways, gene expression, and cellular metabolism. It regulates cyclins and apoptosis-related proteins, thereby inhibiting tumor cell proliferation and inducing apoptosis . This compound also exhibits anti-inflammatory properties by down-regulating inflammation mediators .
Molecular Mechanism
The molecular mechanism of action of this compound involves several key processes. At the molecular level, this compound binds to nuclear receptors FXR and PXR, modulating their activity and influencing gene expression . This binding interaction leads to the down-regulation of bile acid homeostatic genes and the up-regulation of genes involved in lipid metabolism . Additionally, this compound increases the levels of glutathione (GSH) by stimulating γ-glutamylcysteine synthetase, enhancing the antioxidant defense system . These molecular interactions contribute to the diverse biochemical and cellular effects of this compound.
Temporal Effects in Laboratory Settings
In laboratory settings, the effects of this compound have been studied over time to understand its stability, degradation, and long-term effects on cellular function. In vitro studies have shown that this compound can elevate plasma LDL-cholesterol levels by suppressing LDL receptor activity . This effect is observed through post-transcriptional regulation of LDL receptor activity, including decreased binding, uptake, and degradation of LDL . Additionally, this compound has been found to exhibit anti-inflammatory and anti-tumorigenic properties over extended periods .
Dosage Effects in Animal Models
The effects of this compound vary with different dosages in animal models. Studies have shown that this compound increases serum cholesterol levels in mice by suppressing bile acid synthesis . Higher doses of this compound have been associated with increased serum triglyceride levels and alterations in hepatic gene expression involved in lipid metabolism .
Metabolic Pathways
This compound is involved in several metabolic pathways, influencing lipid metabolism and detoxification processes. It modulates fat oxidation, bile acid synthesis, very-low-density lipoprotein (VLDL) production, and lipoprotein concentrations . This compound also interacts with enzymes such as cholesterol 7α-hydroxylase (CYP7A1) and sterol 12α-hydroxylase, which play crucial roles in bile acid synthesis . These interactions contribute to the overall metabolic effects of this compound.
Transport and Distribution
This compound is transported and distributed within cells and tissues through various mechanisms. It is primarily absorbed in the small intestine, with approximately 70% of the compound being absorbed . Once absorbed, this compound undergoes enterohepatic circulation, accumulating in the liver and gastrointestinal tract . This distribution pattern allows this compound to exert its effects on liver metabolism and other biological processes.
Subcellular Localization
The subcellular localization of this compound plays a crucial role in its activity and function. This compound is primarily localized in the cytoplasm and nucleus of cells . It interacts with nuclear receptors FXR and PXR, modulating gene expression and influencing cellular processes . Additionally, this compound may undergo post-translational modifications that direct it to specific compartments or organelles, further enhancing its subcellular localization and activity.
Preparation Methods
Synthetic Routes and Reaction Conditions: Cafestol acetate can be synthesized through the esterification of cafestol with acetic anhydride. The reaction typically involves the use of a catalyst such as pyridine to facilitate the esterification process. The reaction is carried out under reflux conditions, and the product is purified through recrystallization or chromatography techniques .
Industrial Production Methods: In an industrial setting, the production of this compound involves the extraction of cafestol from coffee beans, followed by its esterification. The extraction process includes solvent extraction and purification steps to isolate cafestol. The esterification is then performed on a larger scale using similar reaction conditions as in the laboratory synthesis .
Chemical Reactions Analysis
Types of Reactions: Cafestol acetate undergoes various chemical reactions, including:
Oxidation: this compound can be oxidized to form corresponding ketones or carboxylic acids.
Reduction: Reduction reactions can convert this compound to its alcohol derivatives.
Substitution: Nucleophilic substitution reactions can replace the acetate group with other functional groups.
Common Reagents and Conditions:
Oxidation: Common oxidizing agents include potassium permanganate and chromium trioxide.
Reduction: Reducing agents such as lithium aluminum hydride or sodium borohydride are used.
Substitution: Nucleophiles like hydroxide ions or amines can be employed for substitution reactions.
Major Products:
Oxidation: Formation of ketones or carboxylic acids.
Reduction: Formation of alcohol derivatives.
Substitution: Formation of substituted cafestol derivatives.
Scientific Research Applications
Cafestol acetate has a wide range of scientific research applications, including:
Chemistry: Used as a model compound to study esterification and other organic reactions.
Biology: Investigated for its anti-inflammatory and anti-carcinogenic properties.
Medicine: Potential therapeutic agent for conditions such as cancer and liver diseases.
Industry: Utilized in the development of functional foods and nutraceuticals
Comparison with Similar Compounds
Kahweol: Another diterpene found in coffee beans with similar biological activities but differs in the presence of a conjugated double bond on the furan ring.
Cafestol: The non-esterified form of cafestol acetate, sharing similar pharmacological properties
Uniqueness: this compound is unique due to its esterified form, which may influence its bioavailability and pharmacokinetics compared to non-esterified cafestol. This modification can potentially enhance its therapeutic efficacy and stability .
Properties
CAS No. |
81760-48-7 |
---|---|
Molecular Formula |
C22H30O4 |
Molecular Weight |
358.5 g/mol |
IUPAC Name |
[(1S,4S,12S,13R,16R,17R)-17-hydroxy-12-methyl-8-oxapentacyclo[14.2.1.01,13.04,12.05,9]nonadeca-5(9),6-dien-17-yl]methyl acetate |
InChI |
InChI=1S/C22H30O4/c1-14(23)26-13-22(24)12-21-9-5-17-16-7-10-25-18(16)6-8-20(17,2)19(21)4-3-15(22)11-21/h7,10,15,17,19,24H,3-6,8-9,11-13H2,1-2H3/t15-,17-,19+,20-,21+,22+/m1/s1 |
InChI Key |
PTGGVIKFNQSFBY-WPOGFBIBSA-N |
Isomeric SMILES |
CC(=O)OC[C@]1(C[C@@]23CC[C@@H]4C5=C(CC[C@]4([C@@H]2CC[C@@H]1C3)C)OC=C5)O |
SMILES |
CC(=O)OCC1(CC23CCC4C5=C(CCC4(C2CCC1C3)C)OC=C5)O |
Canonical SMILES |
CC(=O)OCC1(CC23CCC4C5=C(CCC4(C2CCC1C3)C)OC=C5)O |
Synonyms |
cafestol acetate |
Origin of Product |
United States |
Retrosynthesis Analysis
AI-Powered Synthesis Planning: Our tool employs the Template_relevance Pistachio, Template_relevance Bkms_metabolic, Template_relevance Pistachio_ringbreaker, Template_relevance Reaxys, Template_relevance Reaxys_biocatalysis model, leveraging a vast database of chemical reactions to predict feasible synthetic routes.
One-Step Synthesis Focus: Specifically designed for one-step synthesis, it provides concise and direct routes for your target compounds, streamlining the synthesis process.
Accurate Predictions: Utilizing the extensive PISTACHIO, BKMS_METABOLIC, PISTACHIO_RINGBREAKER, REAXYS, REAXYS_BIOCATALYSIS database, our tool offers high-accuracy predictions, reflecting the latest in chemical research and data.
Strategy Settings
Precursor scoring | Relevance Heuristic |
---|---|
Min. plausibility | 0.01 |
Model | Template_relevance |
Template Set | Pistachio/Bkms_metabolic/Pistachio_ringbreaker/Reaxys/Reaxys_biocatalysis |
Top-N result to add to graph | 6 |
Feasible Synthetic Routes
Disclaimer and Information on In-Vitro Research Products
Please be aware that all articles and product information presented on BenchChem are intended solely for informational purposes. The products available for purchase on BenchChem are specifically designed for in-vitro studies, which are conducted outside of living organisms. In-vitro studies, derived from the Latin term "in glass," involve experiments performed in controlled laboratory settings using cells or tissues. It is important to note that these products are not categorized as medicines or drugs, and they have not received approval from the FDA for the prevention, treatment, or cure of any medical condition, ailment, or disease. We must emphasize that any form of bodily introduction of these products into humans or animals is strictly prohibited by law. It is essential to adhere to these guidelines to ensure compliance with legal and ethical standards in research and experimentation.