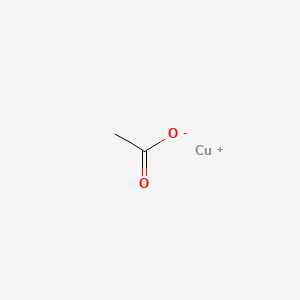
Copper(I) acetate
Overview
Description
Mechanism of Action
Target of Action
Copper(I) acetate, also known as cuprous acetate, primarily targets copper-binding proteins and enzymes in the body . Copper is an essential element in cells and plays a critical role in various cellular physiological and biochemical processes, including energy production and maintenance, antioxidation, enzymatic activity, and signal transduction .
Mode of Action
This compound interacts with its targets by donating or accepting electrons, participating in various reactions . Copper ions are known to reduce fertility when released from copper-containing intrauterine devices . The reaction mechanism for the formation of copper acetate involves the reduction of copper to the Cu1+ form prior to transport .
Biochemical Pathways
This compound affects several biochemical pathways. It is involved in the regulation of cell migration, proliferation, autophagy, DNA damage repair (DDR), and death, as well as in organism development and reproduction . The copper utilization pathways include the CCS-SOD1 route, the Atox1-ATP7A/B-CuPrs pathway, and the Cox17-Sco1/2-CcO route .
Pharmacokinetics
Copper is absorbed from the gut via high affinity copper uptake protein and likely through low affinity copper uptake protein and natural resistance-associated macrophage protein-2 . Once inside the enterocyte, it is bound to copper transport protein ATOX1 which shuttles the ion to copper transporting ATPase-1 on the golgi membrane which take up copper into the golgi apparatus .
Result of Action
The molecular and cellular effects of this compound’s action include the activation of anthracite combustion . Copper acetate increases anthracite reactivity, which was evidenced by decreased onset temperature of combustion and reduced ignition delay time . Copper acetate reduces fuel underburning in the ash residue of anthracite and decreases the amount of CO and NOx in gas-phase products .
Preparation Methods
Synthetic Routes and Reaction Conditions: Copper(I) acetate can be synthesized through several methods:
Direct Reaction: One common method involves the reaction of copper metal with acetic acid. This process typically requires heating to facilitate the reaction.
Reduction Method: Another method involves the reduction of copper(II) acetate with a reducing agent such as hydrogen or a suitable organic compound.
Industrial Production Methods: In industrial settings, cuprous acetate is often produced by the reduction of copper(II) acetate using acetic anhydride in the presence of a catalyst. This method ensures high yield and purity of the product .
Chemical Reactions Analysis
Copper(I) acetate undergoes various chemical reactions, including:
Oxidation: It can be oxidized to copper(II) acetate in the presence of oxidizing agents.
Reduction: It can be reduced to metallic copper using strong reducing agents.
Substitution: this compound reacts with organic halides to form corresponding esters.
Common Reagents and Conditions:
Oxidizing Agents: Oxygen, hydrogen peroxide.
Reducing Agents: Hydrogen, organic reducing agents.
Reaction Conditions: Reactions typically occur under controlled temperatures and pressures to ensure desired outcomes.
Major Products:
Oxidation: Copper(II) acetate.
Reduction: Metallic copper.
Substitution: Organic esters.
Scientific Research Applications
Copper(I) acetate finds applications in various fields:
Biology: It is employed in biochemical studies to investigate copper’s role in biological systems.
Medicine: this compound is explored for its potential antimicrobial properties and its role in drug development.
Industry: It is used in the production of pigments, fungicides, and as a precursor for other copper compounds.
Comparison with Similar Compounds
Copper(I) acetate can be compared with other copper(I) compounds:
Cuprous Oxide (Cu₂O): A red or reddish-brown compound used as a pigment and fungicide.
Cuprous Chloride (Cu₂Cl₂): A whitish to grayish solid used as a catalyst in organic synthesis.
Cuprous Sulfide (Cu₂S): A black compound used in the production of semiconductors.
Uniqueness: this compound is unique due to its specific reactivity with organic halides and its role as a versatile catalyst in organic synthesis .
Properties
IUPAC Name |
copper(1+);acetate | |
---|---|---|
Source | PubChem | |
URL | https://pubchem.ncbi.nlm.nih.gov | |
Description | Data deposited in or computed by PubChem | |
InChI |
InChI=1S/C2H4O2.Cu/c1-2(3)4;/h1H3,(H,3,4);/q;+1/p-1 | |
Source | PubChem | |
URL | https://pubchem.ncbi.nlm.nih.gov | |
Description | Data deposited in or computed by PubChem | |
InChI Key |
RFKZUAOAYVHBOY-UHFFFAOYSA-M | |
Source | PubChem | |
URL | https://pubchem.ncbi.nlm.nih.gov | |
Description | Data deposited in or computed by PubChem | |
Canonical SMILES |
CC(=O)[O-].[Cu+] | |
Source | PubChem | |
URL | https://pubchem.ncbi.nlm.nih.gov | |
Description | Data deposited in or computed by PubChem | |
Molecular Formula |
Cu(CH3COO), C2H3CuO2 | |
Record name | copper(I) acetate | |
Source | Wikipedia | |
URL | https://en.wikipedia.org/wiki/Dictionary_of_chemical_formulas | |
Description | Chemical information link to Wikipedia. | |
Source | PubChem | |
URL | https://pubchem.ncbi.nlm.nih.gov | |
Description | Data deposited in or computed by PubChem | |
DSSTOX Substance ID |
DTXSID0060511 | |
Record name | Acetic acid, copper(1+) salt | |
Source | EPA DSSTox | |
URL | https://comptox.epa.gov/dashboard/DTXSID0060511 | |
Description | DSSTox provides a high quality public chemistry resource for supporting improved predictive toxicology. | |
Molecular Weight |
122.59 g/mol | |
Source | PubChem | |
URL | https://pubchem.ncbi.nlm.nih.gov | |
Description | Data deposited in or computed by PubChem | |
CAS No. |
598-54-9 | |
Record name | Cuprous acetate | |
Source | ChemIDplus | |
URL | https://pubchem.ncbi.nlm.nih.gov/substance/?source=chemidplus&sourceid=0000598549 | |
Description | ChemIDplus is a free, web search system that provides access to the structure and nomenclature authority files used for the identification of chemical substances cited in National Library of Medicine (NLM) databases, including the TOXNET system. | |
Record name | Acetic acid, copper(1+) salt (1:1) | |
Source | EPA Chemicals under the TSCA | |
URL | https://www.epa.gov/chemicals-under-tsca | |
Description | EPA Chemicals under the Toxic Substances Control Act (TSCA) collection contains information on chemicals and their regulations under TSCA, including non-confidential content from the TSCA Chemical Substance Inventory and Chemical Data Reporting. | |
Record name | Acetic acid, copper(1+) salt | |
Source | EPA DSSTox | |
URL | https://comptox.epa.gov/dashboard/DTXSID0060511 | |
Description | DSSTox provides a high quality public chemistry resource for supporting improved predictive toxicology. | |
Record name | Copper(1+) acetate | |
Source | European Chemicals Agency (ECHA) | |
URL | https://echa.europa.eu/substance-information/-/substanceinfo/100.009.036 | |
Description | The European Chemicals Agency (ECHA) is an agency of the European Union which is the driving force among regulatory authorities in implementing the EU's groundbreaking chemicals legislation for the benefit of human health and the environment as well as for innovation and competitiveness. | |
Explanation | Use of the information, documents and data from the ECHA website is subject to the terms and conditions of this Legal Notice, and subject to other binding limitations provided for under applicable law, the information, documents and data made available on the ECHA website may be reproduced, distributed and/or used, totally or in part, for non-commercial purposes provided that ECHA is acknowledged as the source: "Source: European Chemicals Agency, http://echa.europa.eu/". Such acknowledgement must be included in each copy of the material. ECHA permits and encourages organisations and individuals to create links to the ECHA website under the following cumulative conditions: Links can only be made to webpages that provide a link to the Legal Notice page. | |
Record name | CUPROUS ACETATE | |
Source | FDA Global Substance Registration System (GSRS) | |
URL | https://gsrs.ncats.nih.gov/ginas/app/beta/substances/9U3N93LMZB | |
Description | The FDA Global Substance Registration System (GSRS) enables the efficient and accurate exchange of information on what substances are in regulated products. Instead of relying on names, which vary across regulatory domains, countries, and regions, the GSRS knowledge base makes it possible for substances to be defined by standardized, scientific descriptions. | |
Explanation | Unless otherwise noted, the contents of the FDA website (www.fda.gov), both text and graphics, are not copyrighted. They are in the public domain and may be republished, reprinted and otherwise used freely by anyone without the need to obtain permission from FDA. Credit to the U.S. Food and Drug Administration as the source is appreciated but not required. | |
Retrosynthesis Analysis
AI-Powered Synthesis Planning: Our tool employs the Template_relevance Pistachio, Template_relevance Bkms_metabolic, Template_relevance Pistachio_ringbreaker, Template_relevance Reaxys, Template_relevance Reaxys_biocatalysis model, leveraging a vast database of chemical reactions to predict feasible synthetic routes.
One-Step Synthesis Focus: Specifically designed for one-step synthesis, it provides concise and direct routes for your target compounds, streamlining the synthesis process.
Accurate Predictions: Utilizing the extensive PISTACHIO, BKMS_METABOLIC, PISTACHIO_RINGBREAKER, REAXYS, REAXYS_BIOCATALYSIS database, our tool offers high-accuracy predictions, reflecting the latest in chemical research and data.
Strategy Settings
Precursor scoring | Relevance Heuristic |
---|---|
Min. plausibility | 0.01 |
Model | Template_relevance |
Template Set | Pistachio/Bkms_metabolic/Pistachio_ringbreaker/Reaxys/Reaxys_biocatalysis |
Top-N result to add to graph | 6 |
Feasible Synthetic Routes
Disclaimer and Information on In-Vitro Research Products
Please be aware that all articles and product information presented on BenchChem are intended solely for informational purposes. The products available for purchase on BenchChem are specifically designed for in-vitro studies, which are conducted outside of living organisms. In-vitro studies, derived from the Latin term "in glass," involve experiments performed in controlled laboratory settings using cells or tissues. It is important to note that these products are not categorized as medicines or drugs, and they have not received approval from the FDA for the prevention, treatment, or cure of any medical condition, ailment, or disease. We must emphasize that any form of bodily introduction of these products into humans or animals is strictly prohibited by law. It is essential to adhere to these guidelines to ensure compliance with legal and ethical standards in research and experimentation.