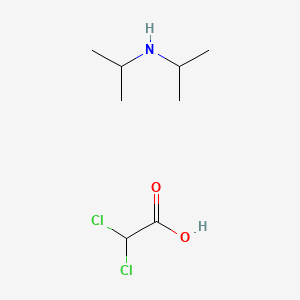
Diisopropylammonium dichloroacetate
Overview
Description
Diisopropylammonium dichloroacetate is an organic compound known for its applications in various chemical processes. It is characterized by its diisopropylammonium and dichloroacetate functional groups. This compound is a white crystalline solid with a slightly bitter taste and is soluble in water, ethanol, and chloroform . It is commonly used as a catalyst and ligand in organic reactions and has significant applications in the medical field, particularly in anti-tumor therapy .
Mechanism of Action
Target of Action
The primary target of Diisopropylammonium dichloroacetate is the enzyme pyruvate dehydrogenase kinase . This enzyme plays a crucial role in the regulation of metabolic processes within the body .
Mode of Action
This compound interacts with its target by inhibiting the activity of pyruvate dehydrogenase kinase . This inhibition leads to an increase in the activity of the pyruvate dehydrogenase complex, promoting the oxidation of lactate and reducing morbidity in acquired and congenital forms of lactic acidosis .
Biochemical Pathways
This compound affects multiple biochemical pathways. It stimulates peripheral glucose utilization and inhibits gluconeogenesis, thereby reducing hyperglycemia in animals and humans with diabetes mellitus . It also inhibits lipogenesis and cholesterolgenesis, thereby decreasing circulating lipid and lipoprotein levels .
Pharmacokinetics
It is known that the compound is easily soluble in water, ethanol, or chloroform, and slightly soluble in ether . This solubility profile may influence its absorption, distribution, metabolism, and excretion (ADME) properties, and thus its bioavailability.
Result of Action
The molecular and cellular effects of this compound’s action are diverse. It improves the energy metabolism of hepatocytes, promotes the regeneration of injured hepatocytes, increases the rate of tissue cell respiration and oxygen respiration, and reduces the accumulation of fat in the liver .
Action Environment
Environmental factors can influence the action, efficacy, and stability of this compound. For instance, the compound appears to inhibit its own metabolism, which may influence the duration of its pharmacologic actions and lead to toxicity . Furthermore, the compound can cause a reversible peripheral neuropathy that may be related to thiamine deficiency and may be ameliorated or prevented with thiamine supplementation .
Biochemical Analysis
Biochemical Properties
Diisopropylammonium dichloroacetate plays a crucial role in biochemical reactions by interacting with various enzymes, proteins, and biomolecules. It is known to inhibit the enzyme pyruvate dehydrogenase kinase, which leads to the activation of the pyruvate dehydrogenase complex . This interaction enhances the conversion of pyruvate to acetyl-CoA, thereby promoting glucose oxidation over glycolysis. Additionally, this compound interacts with the enzyme HMG CoA reductase, inhibiting cholesterol biosynthesis . These interactions highlight the compound’s role in regulating metabolic pathways and cellular energy production.
Cellular Effects
This compound exerts various effects on different cell types and cellular processes. It has been shown to influence cell signaling pathways, gene expression, and cellular metabolism. For instance, this compound treatment induces changes in the cell-cycle profiles of glioblastoma cells, leading to an increase in the proportion of cells in the G2–M phase . This compound also affects glucose and fat metabolism in diabetic and normal tissues, promoting glucose oxidation and inhibiting fatty acid oxidation . These cellular effects underscore its potential therapeutic applications in metabolic disorders and cancer treatment.
Molecular Mechanism
The molecular mechanism of this compound involves several key interactions at the molecular level. It inhibits pyruvate dehydrogenase kinase, leading to the activation of the pyruvate dehydrogenase complex and increased glucose oxidation . Additionally, this compound inhibits HMG CoA reductase, reducing cholesterol biosynthesis . These interactions result in significant changes in cellular metabolism, including enhanced glucose utilization and reduced lipid synthesis. The compound’s ability to modulate these pathways makes it a promising candidate for therapeutic interventions.
Temporal Effects in Laboratory Settings
In laboratory settings, the effects of this compound have been observed to change over time. The compound exhibits stability under various conditions, with a shelf life of up to three years when stored at -20°C . In vitro studies have shown that this compound can exert prolonged hypoglycemic effects in diabetic rats, indicating its potential for long-term therapeutic applications . Additionally, the compound’s stability and degradation profile suggest that it can maintain its biochemical activity over extended periods.
Dosage Effects in Animal Models
The effects of this compound vary with different dosages in animal models. Studies have shown that the compound exerts a significant hypoglycemic effect in diabetic rats at specific dosages, while higher doses may lead to adverse effects . For instance, this compound administration in depancreatized dogs resulted in a reduction in blood glucose levels and hepatic glucose supply . Chronic administration of high doses may lead to potential toxicity and accumulation of the compound . These findings highlight the importance of optimizing dosage to achieve therapeutic benefits while minimizing adverse effects.
Metabolic Pathways
This compound is involved in several metabolic pathways, including glucose and lipid metabolism. It stimulates peripheral glucose utilization and inhibits gluconeogenesis, thereby reducing hyperglycemia in diabetic animals . The compound also inhibits lipogenesis and cholesterolgenesis, leading to decreased circulating lipid and lipoprotein levels . By activating the pyruvate dehydrogenase complex, this compound facilitates the oxidation of lactate and improves cardiac output under conditions of myocardial ischemia . These metabolic effects underscore its potential for treating metabolic disorders.
Transport and Distribution
The transport and distribution of this compound within cells and tissues involve various transporters and binding proteins. The compound is known to be hygroscopic and soluble in DMSO, methanol, and ethanol . Its distribution within tissues is influenced by its solubility and interaction with cellular transporters. Studies have shown that this compound can be effectively transported to target tissues, where it exerts its biochemical effects . Understanding its transport and distribution mechanisms is crucial for optimizing its therapeutic applications.
Subcellular Localization
The subcellular localization of this compound plays a significant role in its activity and function. The compound is known to localize within specific cellular compartments, where it interacts with target enzymes and proteins. For instance, this compound localizes to the mitochondria, where it inhibits pyruvate dehydrogenase kinase and activates the pyruvate dehydrogenase complex . This localization is essential for its role in regulating cellular metabolism and energy production. Additionally, the compound’s interaction with HMG CoA reductase occurs within the endoplasmic reticulum, further highlighting its subcellular specificity .
Preparation Methods
Diisopropylammonium dichloroacetate can be synthesized through two primary methods:
Acylation of Isopropylamine with Dichloroacetic Acid: This method involves the acylation of isopropylamine with dichloroacetic acid, followed by neutralization to obtain the target product.
Direct Reaction of Dichloroacetic Acid with Isopropylamine: In this method, dichloroacetic acid reacts directly with isopropylamine under acidic conditions to produce this compound.
Chemical Reactions Analysis
Diisopropylammonium dichloroacetate undergoes various chemical reactions, including:
Oxidation: It can be oxidized under specific conditions to form different products.
Reduction: The compound can be reduced using common reducing agents.
Substitution: It undergoes substitution reactions, particularly with nucleophiles.
Common Reagents and Conditions: Typical reagents include oxidizing agents like potassium permanganate and reducing agents like sodium borohydride.
Major Products: The major products formed depend on the type of reaction and the reagents used.
Scientific Research Applications
Diisopropylammonium dichloroacetate has a wide range of scientific research applications:
Medicine: It has shown promising results in anti-tumor therapy, particularly in non-small cell lung cancer.
Industry: The compound is used in the synthesis of other chemicals and as a plant growth regulator.
Comparison with Similar Compounds
Diisopropylammonium dichloroacetate is unique compared to other similar compounds due to its specific functional groups and applications:
Properties
CAS No. |
660-27-5 |
---|---|
Molecular Formula |
C8H17Cl2NO2 |
Molecular Weight |
230.13 g/mol |
IUPAC Name |
2,2-dichloroacetate;di(propan-2-yl)azanium |
InChI |
InChI=1S/C6H15N.C2H2Cl2O2/c1-5(2)7-6(3)4;3-1(4)2(5)6/h5-7H,1-4H3;1H,(H,5,6) |
InChI Key |
ILKBHIBYKSHTKQ-UHFFFAOYSA-N |
SMILES |
CC(C)NC(C)C.C(C(=O)O)(Cl)Cl |
Canonical SMILES |
CC(C)[NH2+]C(C)C.C(C(=O)[O-])(Cl)Cl |
660-27-5 | |
Pictograms |
Irritant |
Synonyms |
di-isopropylamine dichloroacetate diisopropylamine dichloroacetate diisopropylammonium dichloroethanoate DIPA dipromonium |
Origin of Product |
United States |
Retrosynthesis Analysis
AI-Powered Synthesis Planning: Our tool employs the Template_relevance Pistachio, Template_relevance Bkms_metabolic, Template_relevance Pistachio_ringbreaker, Template_relevance Reaxys, Template_relevance Reaxys_biocatalysis model, leveraging a vast database of chemical reactions to predict feasible synthetic routes.
One-Step Synthesis Focus: Specifically designed for one-step synthesis, it provides concise and direct routes for your target compounds, streamlining the synthesis process.
Accurate Predictions: Utilizing the extensive PISTACHIO, BKMS_METABOLIC, PISTACHIO_RINGBREAKER, REAXYS, REAXYS_BIOCATALYSIS database, our tool offers high-accuracy predictions, reflecting the latest in chemical research and data.
Strategy Settings
Precursor scoring | Relevance Heuristic |
---|---|
Min. plausibility | 0.01 |
Model | Template_relevance |
Template Set | Pistachio/Bkms_metabolic/Pistachio_ringbreaker/Reaxys/Reaxys_biocatalysis |
Top-N result to add to graph | 6 |
Feasible Synthetic Routes
Disclaimer and Information on In-Vitro Research Products
Please be aware that all articles and product information presented on BenchChem are intended solely for informational purposes. The products available for purchase on BenchChem are specifically designed for in-vitro studies, which are conducted outside of living organisms. In-vitro studies, derived from the Latin term "in glass," involve experiments performed in controlled laboratory settings using cells or tissues. It is important to note that these products are not categorized as medicines or drugs, and they have not received approval from the FDA for the prevention, treatment, or cure of any medical condition, ailment, or disease. We must emphasize that any form of bodily introduction of these products into humans or animals is strictly prohibited by law. It is essential to adhere to these guidelines to ensure compliance with legal and ethical standards in research and experimentation.