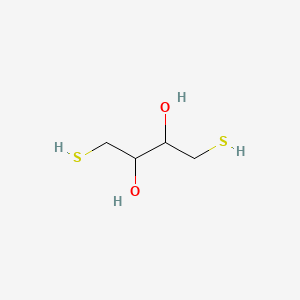
Dithiothreitol
- Click on QUICK INQUIRY to receive a quote from our team of experts.
- With the quality product at a COMPETITIVE price, you can focus more on your research.
Overview
Description
Dithiothreitol is an organosulfur compound with the chemical formula C4H10O2S2. It is a colorless compound classified as both a dithiol and a diol. This compound is commonly used as a reducing agent in biochemical research and is also known as Cleland’s reagent, named after W. Wallace Cleland. The compound’s name is derived from the four-carbon sugar, threose .
Preparation Methods
Synthetic Routes and Reaction Conditions: Dithiothreitol can be synthesized through the reduction of 2,3-O-isopropylidene this compound using sodium hydroxide solution. The reaction involves stirring the mixture for several hours, followed by extraction with ethyl acetate and distillation under reduced pressure to obtain the final product .
Industrial Production Methods: In industrial settings, this compound is produced by dissolving the compound in water to create a solution. For example, a 1 M solution can be prepared by dissolving 3.86 grams of this compound in water to a final volume of 25 milliliters . The solution is then stored at low temperatures to maintain stability.
Chemical Reactions Analysis
Types of Reactions: Dithiothreitol primarily undergoes reduction reactions. It is a strong reducing agent with a redox potential of -0.33 V at pH 7. The reduction of disulfide bonds by this compound involves two sequential thiol-disulfide exchange reactions, resulting in the formation of a stable six-membered ring with an internal disulfide bond .
Common Reagents and Conditions: this compound is often used in aqueous solutions at concentrations ranging from 1 mM to 100 mM. The reduction reactions typically occur at pH values above 7, as the thiolate form of this compound is more reactive than the protonated thiol form .
Major Products Formed: The primary product of the reduction of disulfide bonds by this compound is the formation of reduced disulfide bonds and oxidized this compound, which forms a stable six-membered ring structure .
Scientific Research Applications
Dithiothreitol has a wide range of applications in scientific research, including:
Chemistry: It is used as a reducing agent to break down disulfide bonds in proteins and other molecules.
Medicine: It is used in the preparation of thiolated DNA for biosensors and other diagnostic applications.
Industry: this compound is used in the production of biofuels and as an antioxidant agent.
Mechanism of Action
Dithiothreitol exerts its effects by reducing disulfide bonds to dithiols. This process involves two sequential thiol-disulfide exchange reactions, resulting in the formation of a stable six-membered ring with an internal disulfide bond. The thiolate form of this compound is the active species in these reactions, and its reducing power is limited to pH values above 7 .
Comparison with Similar Compounds
Dithioerythritol: An epimeric compound of dithiothreitol, also used as a reducing agent.
Tris(2-carboxyethyl)phosphine (TCEP): A sulfhydryl reductant that is more stable than this compound and can be used in a wider range of pH conditions.
Uniqueness: this compound is unique due to its high conformational propensity to form a six-membered ring with an internal disulfide bond, making it an unusually strong reducing agent. Its ability to prevent the formation of disulfide bonds in proteins and other molecules makes it a valuable tool in biochemical research .
Properties
CAS No. |
7634-42-6 |
---|---|
Molecular Formula |
C4H10O2S2 |
Molecular Weight |
154.3 g/mol |
IUPAC Name |
1,4-bis(sulfanyl)butane-2,3-diol |
InChI |
InChI=1S/C4H10O2S2/c5-3(1-7)4(6)2-8/h3-8H,1-2H2 |
InChI Key |
VHJLVAABSRFDPM-UHFFFAOYSA-N |
impurities |
Oxidized form: <2.5% (absorbance at 283nm) |
SMILES |
C(C(C(CS)O)O)S |
Canonical SMILES |
C(C(C(CS)O)O)S |
boiling_point |
BP: 115-116 °C at 1 mm Hg Can be sublimed at 37 °C and 0.005 mm pressure. BP: 125-130 at 2 mm Hg |
Color/Form |
Needles from ether Solid |
melting_point |
42-43 °C |
3483-12-3 7634-42-6 6892-68-8 16096-97-2 27565-41-9 |
|
physical_description |
Slightly hygroscopic solid; [Merck Index] White powder; [Sigma-Aldrich MSDS] White powder with an unpleasant odor; [Alfa Aesar MSDS] |
Pictograms |
Irritant |
shelf_life |
Chemical stability: May decompose on exposure to moist air or water. Stable under recommended storage conditions. |
solubility |
Freely soluble in water Freely soluble in ethanol, acetone, ethyl acetate, chloroform, ethe |
Synonyms |
Cleland Reagent Cleland's Reagent Clelands Reagent Dithiothreitol Reagent, Cleland Reagent, Cleland's Sputolysin |
vapor_pressure |
1.28X10-4 mm Hg at 25 °C (est) |
Origin of Product |
United States |
Retrosynthesis Analysis
AI-Powered Synthesis Planning: Our tool employs the Template_relevance Pistachio, Template_relevance Bkms_metabolic, Template_relevance Pistachio_ringbreaker, Template_relevance Reaxys, Template_relevance Reaxys_biocatalysis model, leveraging a vast database of chemical reactions to predict feasible synthetic routes.
One-Step Synthesis Focus: Specifically designed for one-step synthesis, it provides concise and direct routes for your target compounds, streamlining the synthesis process.
Accurate Predictions: Utilizing the extensive PISTACHIO, BKMS_METABOLIC, PISTACHIO_RINGBREAKER, REAXYS, REAXYS_BIOCATALYSIS database, our tool offers high-accuracy predictions, reflecting the latest in chemical research and data.
Strategy Settings
Precursor scoring | Relevance Heuristic |
---|---|
Min. plausibility | 0.01 |
Model | Template_relevance |
Template Set | Pistachio/Bkms_metabolic/Pistachio_ringbreaker/Reaxys/Reaxys_biocatalysis |
Top-N result to add to graph | 6 |
Feasible Synthetic Routes
Disclaimer and Information on In-Vitro Research Products
Please be aware that all articles and product information presented on BenchChem are intended solely for informational purposes. The products available for purchase on BenchChem are specifically designed for in-vitro studies, which are conducted outside of living organisms. In-vitro studies, derived from the Latin term "in glass," involve experiments performed in controlled laboratory settings using cells or tissues. It is important to note that these products are not categorized as medicines or drugs, and they have not received approval from the FDA for the prevention, treatment, or cure of any medical condition, ailment, or disease. We must emphasize that any form of bodily introduction of these products into humans or animals is strictly prohibited by law. It is essential to adhere to these guidelines to ensure compliance with legal and ethical standards in research and experimentation.