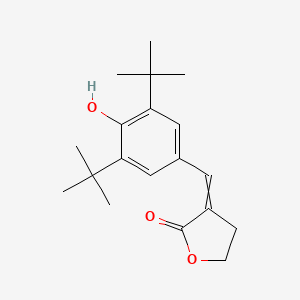
Pgs-IN-1
Overview
Description
PGS-IN-1 (KME-4) is a dual inhibitor targeting prostaglandin synthetase (PGS) and 5-lipoxygenase (5-LOX), with IC50 values of 0.28 μM and 1.05 μM, respectively . It belongs to a class of compounds designed to modulate inflammatory pathways by simultaneously blocking prostaglandin and leukotriene biosynthesis. Unlike selective cyclooxygenase (COX) inhibitors, this compound's dual mechanism may reduce side effects associated with traditional non-steroidal anti-inflammatory drugs (NSAIDs), such as gastrointestinal toxicity .
Q & A
Basic Research Questions
Q. What is the primary biochemical mechanism of Pgs-IN-1, and how should researchers validate its inhibitory effects experimentally?
this compound is a dual inhibitor of prostaglandin synthetase (IC50 = 0.28 μM) and 5-lipoxygenase (IC50 = 1.05 μM). To validate its activity, use cell-free enzyme inhibition assays with purified COX/LOX isoforms. Include positive controls like Phenidone (a known dual COX/LOX inhibitor) and measure inhibition kinetics via fluorometric or spectrophotometric methods. Ensure replicates (n ≥ 3) and report both IC50 and selectivity ratios .
Q. How should researchers design dose-response experiments for this compound to ensure reproducibility?
- Concentration range : Test 0.1–10 μM (spanning its IC50 values).
- Controls : Vehicle (e.g., DMSO ≤ 0.1%), positive inhibitors (e.g., Flurbiprofen for COX), and baseline enzyme activity.
- Assay conditions : Standardize pH, temperature, and substrate concentrations (e.g., arachidonic acid for COX/LOX).
- Data analysis : Use nonlinear regression (e.g., GraphPad Prism) to calculate IC50 and Hill slopes. Report coefficient of variation (CV) for replicates .
Q. What are the critical parameters for evaluating this compound’s selectivity between COX and 5-LOX pathways?
- Enzyme source : Use homologous systems (e.g., human recombinant enzymes) to avoid species-specific biases.
- Parallel assays : Run COX (prostaglandin E2 ELISA) and 5-LOX (LTB4 quantification via HPLC) assays under identical conditions.
- Selectivity index : Calculate as IC50(5-LOX)/IC50(COX). A value >3 indicates preferential COX inhibition .
Advanced Research Questions
Q. How can researchers resolve contradictions in reported IC50 values of this compound across studies?
Discrepancies may arise from:
- Enzyme purity : Contaminants in commercial enzyme preparations.
- Assay interference : Fluorescent probes (e.g., DCFH-DA) interacting with this compound. Methodology :
- Validate assays with orthogonal techniques (e.g., LC-MS for metabolite quantification).
- Compare results against standardized reference inhibitors.
- Use the FINER framework to assess experimental feasibility and novelty .
Q. What computational strategies are effective for optimizing this compound’s pharmacokinetic profile while retaining dual inhibition?
- Molecular docking : Screen against COX-1/2 and 5-LOX crystal structures (PDB IDs: 3LN1, 3V92) to identify binding hotspots.
- ADMET prediction : Use tools like SwissADME to optimize logP (target 2–3) and reduce CYP450 inhibition.
- Analog synthesis : Introduce polar groups (e.g., -OH, -COOH) to improve solubility without disrupting key hydrophobic interactions .
Q. How should researchers integrate this compound into in vivo inflammation models to assess therapeutic potential?
- Model selection : Use rodent models of adjuvant-induced arthritis (for COX) or zymosan-induced peritonitis (for 5-LOX).
- Dosing regimen : Administer this compound intraperitoneally (1–10 mg/kg) with pharmacokinetic profiling (Tmax, Cmax).
- Outcome metrics : Quantify prostaglandins (PGE2) and leukotrienes (LTB4) in plasma/tissue via ELISA.
- Ethical alignment : Follow ARRIVE guidelines for humane endpoints and sample size justification .
Q. Methodological Frameworks
Q. How can the PICO framework structure hypothesis-driven studies on this compound?
- Population : In vitro (e.g., RAW 264.7 macrophages) or in vivo (e.g., Sprague-Dawley rats).
- Intervention : this compound at IC50-equivalent doses.
- Comparison : NSAIDs (e.g., Indomethacin) or LOX inhibitors (e.g., Zileuton).
- Outcome : Inhibition efficiency (%), cytokine/mediator levels.
- Time : Acute (24–48 hr) vs. chronic (7–14 days) exposure .
Q. What statistical approaches are robust for analyzing this compound’s dose-dependent effects?
- ANOVA with post hoc tests : Compare means across dose groups.
- Bland-Altman plots : Assess agreement between technical replicates.
- Power analysis : Ensure n ≥ 6 for in vivo studies (α = 0.05, β = 0.2).
- Meta-analysis : Pool data from multiple studies using random-effects models to address variability .
Comparison with Similar Compounds
Key Compounds for Comparison
Licofelone (ML-3000)
Phenidone
PF-4693627
Zileuton
Comparative Analysis
2.1 Target Selectivity and Potency
Compound | Primary Targets | IC50 (PGS/mPGES-1) | IC50 (5-LOX/COX) | Selectivity Profile |
---|---|---|---|---|
PGS-IN-1 | PGS, 5-LOX | 0.28 μM (PGS) | 1.05 μM (5-LOX) | Dual PGS/5-LOX inhibitor |
Licofelone | COX-1, COX-2, 5-LOX | N/A | 0.18 μM (5-LOX), 0.21 μM (COX) | Triple inhibitor (COX-1/COX-2/5-LOX) |
Phenidone | COX, 5-LOX | N/A | N/A | Broad-spectrum COX/LOX inhibitor |
PF-4693627 | mPGES-1 | 3 nM (mPGES-1) | N/A | Highly selective for mPGES-1 |
Zileuton | 5-LOX | N/A | ~0.5–1.0 μM (5-LOX) | Selective 5-LOX inhibitor |
- This compound vs. Licofelone : Licofelone exhibits higher potency (IC50 = 0.18 μM for 5-LOX vs. 1.05 μM for this compound) and targets both COX isoforms and 5-LOX, making it a more comprehensive anti-inflammatory agent . However, this compound’s inhibition of PGS (a broader enzyme family) may influence a wider range of prostaglandins.
- This compound vs. PF-4693627 : PF-4693627 is 1,000-fold more potent against mPGES-1 (IC50 = 3 nM) but lacks 5-LOX activity. This selectivity makes PF-4693627 preferable for conditions driven by prostaglandin E2 (PGE2), such as osteoarthritis .
- This compound vs. Phenidone : Phenidone’s dual COX/LOX inhibition is less characterized in terms of IC50 but demonstrates in vivo efficacy in hypertension and autoimmune encephalomyelitis models . This compound’s quantified potency provides a clearer preclinical profile.
- This compound vs. Zileuton : Zileuton is a selective 5-LOX inhibitor (IC50 ~0.5–1.0 μM), comparable to this compound’s 5-LOX activity. However, Zileuton lacks PGS inhibition, limiting its utility in prostaglandin-driven pathologies .
2.3 Advantages and Limitations
- This compound: Advantages: Dual inhibition avoids complete COX suppression (reducing GI toxicity). Broad PGS targeting may modulate multiple prostaglandins.
- Licofelone :
- PF-4693627 :
- Advantages: High selectivity and oral availability.
- Limitations: Narrow focus on mPGES-1 limits utility in leukotriene-driven diseases .
Preparation Methods
Conventional Polycondensation Synthesis
The traditional method for PGS synthesis involves a two-step polycondensation process. First, glycerol and sebacic acid are combined in stoichiometric ratios (typically 1:1) and heated under inert gas (nitrogen or argon) at 120–150°C for 24–48 hours to form a prepolymer . This prepolymer is then cross-linked under vacuum (40–100 mTorr) at elevated temperatures (130–150°C) for an additional 24–72 hours .
Reaction Kinetics and Parameter Optimization
Studies demonstrate that the activation energy of polycondensation decreases with equimolar glycerol-to-sebacic acid ratios, favoring esterification . For example, Matyszczak et al. (2020) observed first-order kinetics during initial stages, transitioning to diffusion-controlled mechanisms as viscosity increased . Gadomska-Gajadhur et al. (2018) optimized prepolymer synthesis using a Box–Behnken design, identifying 150°C for 5 hours under argon as ideal for maximizing esterification (82%) and carboxylic group conversion (89%) .
Table 1: Conventional Synthesis Parameters and Outcomes
Microwave-Assisted Synthesis
Microwave-assisted synthesis (MwAS) reduces prepolymerization time from days to minutes. Aydin et al. (2013) achieved PGS synthesis in 3 minutes at 180°C without catalysts or vacuum, yielding a Young’s modulus of 0.50 MPa . However, this method caused glycerol loss due to boiling, altering the final G:S ratio to 0.22:0.78 . Recent advances by Riaz et al. (2022) utilized nitrogen flow during MwAS to minimize monomer evaporation, achieving homogeneous prepolymers in 3 hours .
Enzymatic Synthesis
Enzymatic methods offer eco-friendly alternatives by using lipases (e.g., Candida antarctica) to catalyze esterification at milder temperatures (60–80°C). Valerio et al. (2018) reported 70% esterification efficiency at 70°C, though the process required longer durations (72 hours) compared to conventional methods . Enzymatic PGS exhibits lower cross-linking density, making it suitable for soft tissue applications requiring high elasticity .
Impact of Atmospheric Conditions
Martín-Cabezuelo et al. (2021a) demonstrated that oxidative atmospheres (dry air) during prepolymerization increase branching via secondary hydroxyl oxidation, while inert gases (argon) favor linear chain growth . PGS synthesized under argon showed a 40% higher tensile strength than air-cured variants, highlighting atmospheric control as a critical parameter .
Cross-Linking and Post-Synthesis Modifications
Cross-linking methods, such as photopolymerization and urethane bonding, enhance PGS stability. Liu et al. (2007a) developed thermoplastic PGS (TM-PGS) by hot-pressing prepolymers at 130°C and 15 MPa, achieving a glass transition temperature (Tg) of −40°C . UV-induced cross-linking with photoinitiators (e.g., Irgacure 2959) reduced curing time to 10 minutes, enabling rapid prototyping of microfluidic devices .
Degradation and Biocompatibility
PGS degradation is hydrolytic, with rates adjustable via cross-linking density. In vitro studies show 50–90% mass loss over 8 weeks in phosphate-buffered saline, while in vivo models (rat subcutaneous implants) exhibit complete absorption within 12 weeks . Cytotoxicity assays confirm >90% cell viability for PGS with glycerol excess (G:S = 1.2:1), making it ideal for direct tissue contact .
Properties
Molecular Formula |
C19H26O3 |
---|---|
Molecular Weight |
302.4 g/mol |
IUPAC Name |
3-[(3,5-ditert-butyl-4-hydroxyphenyl)methylidene]oxolan-2-one |
InChI |
InChI=1S/C19H26O3/c1-18(2,3)14-10-12(9-13-7-8-22-17(13)21)11-15(16(14)20)19(4,5)6/h9-11,20H,7-8H2,1-6H3 |
InChI Key |
DFPYHQJPGCODSB-UHFFFAOYSA-N |
Canonical SMILES |
CC(C)(C)C1=CC(=CC(=C1O)C(C)(C)C)C=C2CCOC2=O |
Synonyms |
alpha-(3,5-di-tert-butyl-4-hydroxybenzylidene)gamma-butyrolactone KME 4 KME-4 |
Origin of Product |
United States |
Synthesis routes and methods
Procedure details
Disclaimer and Information on In-Vitro Research Products
Please be aware that all articles and product information presented on BenchChem are intended solely for informational purposes. The products available for purchase on BenchChem are specifically designed for in-vitro studies, which are conducted outside of living organisms. In-vitro studies, derived from the Latin term "in glass," involve experiments performed in controlled laboratory settings using cells or tissues. It is important to note that these products are not categorized as medicines or drugs, and they have not received approval from the FDA for the prevention, treatment, or cure of any medical condition, ailment, or disease. We must emphasize that any form of bodily introduction of these products into humans or animals is strictly prohibited by law. It is essential to adhere to these guidelines to ensure compliance with legal and ethical standards in research and experimentation.