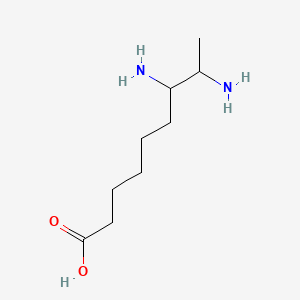
7,8-Diaminononanoic acid
Overview
Description
7,8-Diaminononanoic acid is an amino fatty acid with the molecular formula C₉H₂₀N₂O₂. It carries amino substituents at positions 7 and 8 on the nonanoic acid chain. This compound is notable for its role as an intermediate in the biosynthesis of biotin, a vital coenzyme in various metabolic processes .
Preparation Methods
Synthetic Routes and Reaction Conditions: The synthesis of 7,8-diaminononanoic acid typically involves the selective introduction of amino groups at the 7th and 8th positions of nonanoic acid. One common method is the catalytic hydrogenation of 7,8-dinitrononanoic acid, which can be prepared by nitration of nonanoic acid. The hydrogenation process is usually carried out under mild conditions using a palladium catalyst in the presence of hydrogen gas .
Industrial Production Methods: Industrial production of this compound often employs similar synthetic routes but on a larger scale. The process involves the use of high-pressure reactors and continuous flow systems to ensure efficient hydrogenation and high yield of the desired product .
Chemical Reactions Analysis
Types of Reactions: 7,8-Diaminononanoic acid undergoes various chemical reactions, including:
Oxidation: The amino groups can be oxidized to form corresponding nitroso or nitro derivatives.
Reduction: The carboxylic acid group can be reduced to an alcohol using reducing agents like lithium aluminum hydride.
Substitution: The amino groups can participate in nucleophilic substitution reactions, forming derivatives such as amides or ureas.
Common Reagents and Conditions:
Oxidation: Reagents like potassium permanganate or hydrogen peroxide under acidic conditions.
Reduction: Lithium aluminum hydride in anhydrous ether.
Substitution: Acyl chlorides or isocyanates in the presence of a base.
Major Products:
Oxidation: 7,8-dinitrononanoic acid.
Reduction: 7,8-diamino-1-nonanol.
Substitution: 7,8-diaminononanamide or 7,8-diaminononylurea.
Scientific Research Applications
Biochemical Significance
7,8-Diaminononanoic acid is a key intermediate in the biosynthesis of biotin. It is synthesized from pimeloyl-CoA through a series of enzymatic reactions involving transaminases and other enzymes. The following sections detail its applications in various research domains.
Biocatalysis
This compound is involved in several enzymatic reactions:
- Dethiobiotin Synthetase : This enzyme catalyzes the carbonylation of this compound to form dethiobiotin. The reaction proceeds regiospecifically via the N7-carbamate intermediate, highlighting its importance in biotin biosynthesis .
- Transaminase Activity : The compound acts as a substrate for aminotransferases that utilize S-adenosyl-L-methionine as an amino donor to convert 8-amino-7-oxononanoate into 7,8-diaminopelargonic acid .
Antimicrobial and Herbicide Development
Research has identified this compound as a target for developing antimicrobial agents. Its structural properties allow it to inhibit the growth of certain bacteria by disrupting their metabolic pathways related to biotin synthesis .
- Antimicrobial Activity : Studies have shown that derivatives of this compound exhibit significant antimicrobial properties against various pathogens, making them potential candidates for new antibiotic formulations .
Metabolic Pathways
The compound plays a crucial role in metabolic pathways within microorganisms such as Escherichia coli. It is produced during the metabolism of fatty acids and is involved in the synthesis of biotin precursors .
Table 1: Key Enzymatic Reactions Involving this compound
Potential Therapeutic Applications
Due to its role in biotin synthesis and microbial metabolism, there is ongoing research into the therapeutic potential of targeting pathways involving this compound for treating bacterial infections and metabolic disorders .
Case Study 1: Biocatalysis Optimization
A study investigated the efficiency of Corynebacterium amycolatum enzymes in utilizing various fatty acids to produce this compound. The findings indicated that engineered strains could enhance yields significantly compared to wild-type strains .
Case Study 2: Antimicrobial Efficacy
Research conducted on derivatives of this compound demonstrated effective inhibition against Mycobacterium tuberculosis, suggesting its potential use in developing new anti-tubercular drugs .
Mechanism of Action
The primary mechanism of action of 7,8-diaminononanoic acid involves its role as a substrate in the biosynthesis of biotin. The compound undergoes ATP-dependent carboxylation, catalyzed by the enzyme dethiobiotin synthetase, to form an ureido ring. This reaction is crucial for the formation of biotin, which acts as a coenzyme in carboxylation reactions essential for fatty acid synthesis and amino acid metabolism .
Comparison with Similar Compounds
- 7,8-Diaminopelargonic acid
- 7,8-Diaminoheptanoic acid
- 7,8-Diaminooctanoic acid
Comparison: 7,8-Diaminononanoic acid is unique due to its specific role in biotin synthesis. While similar compounds like 7,8-diaminopelargonic acid also participate in metabolic pathways, this compound’s position in the nonanoic acid chain and its specific enzymatic interactions make it distinct. Its longer carbon chain compared to heptanoic and octanoic acids provides different physical and chemical properties, influencing its reactivity and applications .
Biological Activity
7,8-Diaminononanoic acid (DAPA), also known as 7,8-diaminopelargonic acid, is an amino fatty acid that plays a crucial role in various biological processes, particularly in the biosynthesis of biotin. This compound is characterized by its medium-chain fatty acid structure, containing a total of nine carbon atoms and two amino groups at the 7th and 8th positions. Its chemical formula is with a molecular weight of approximately 188.27 g/mol.
- IUPAC Name : this compound
- CAS Number : 21738-21-6
- Molecular Weight : 188.2673 g/mol
- Chemical Structure :
Biological Role
This compound is primarily recognized for its involvement in the biosynthesis of biotin, an essential vitamin for various metabolic processes. It serves as an intermediate in the pathway that converts simple precursors into biotin through a series of enzymatic reactions.
Enzymatic Reactions
-
Dethiobiotin Synthetase Reaction :
- Reaction :
- Enzyme : Dethiobiotin synthetase (BIO4)
- Function : Catalyzes the formation of dethiobiotin from DAPA.
-
Adenosylmethionine-8-amino-7-oxononanoate Aminotransferase Reaction :
- Reaction :
- Enzyme : Adenosylmethionine-8-amino-7-oxononanoate aminotransferase (BIO3)
- Function : Transfers an amino group to form DAPA from its precursor.
Biological Activity and Effects
The biological activity of this compound can be summarized as follows:
- Role in Biotin Biosynthesis : DAPA is a key precursor in the synthesis of biotin, which is vital for carboxylation reactions and plays a significant role in energy metabolism.
- Antimicrobial Properties : Research indicates that some derivatives of DAPA exhibit antimicrobial activity, suggesting potential applications in developing new antibiotics or herbicides .
Case Studies and Research Findings
Several studies have investigated the biological activity and applications of this compound:
- Biotin Biosynthesis Pathway Analysis :
- Antimicrobial Activity Assessment :
- Metabolic Pathway Exploration :
Data Tables
Property | Value |
---|---|
Chemical Formula | C9H20N2O2 |
Molecular Weight | 188.2673 g/mol |
CAS Number | 21738-21-6 |
Role | Biotin biosynthesis precursor |
Solubility | Slightly soluble in water |
Enzyme | Reaction | Function |
---|---|---|
Dethiobiotin Synthetase | ATP + 7,8-diaminononanoate + CO₂ → ADP + phosphate + dethiobiotin | Converts DAPA to dethiobiotin |
Adenosylmethionine Aminotransferase | S-adenosyl-L-methionine + 8-amino-7-oxononanoate → S-adeno... + 7,8-diaminononanoate | Forms DAPA from its precursor |
Properties
IUPAC Name |
7,8-diaminononanoic acid | |
---|---|---|
Source | PubChem | |
URL | https://pubchem.ncbi.nlm.nih.gov | |
Description | Data deposited in or computed by PubChem | |
InChI |
InChI=1S/C9H20N2O2/c1-7(10)8(11)5-3-2-4-6-9(12)13/h7-8H,2-6,10-11H2,1H3,(H,12,13) | |
Source | PubChem | |
URL | https://pubchem.ncbi.nlm.nih.gov | |
Description | Data deposited in or computed by PubChem | |
InChI Key |
KCEGBPIYGIWCDH-UHFFFAOYSA-N | |
Source | PubChem | |
URL | https://pubchem.ncbi.nlm.nih.gov | |
Description | Data deposited in or computed by PubChem | |
Canonical SMILES |
CC(C(CCCCCC(=O)O)N)N | |
Source | PubChem | |
URL | https://pubchem.ncbi.nlm.nih.gov | |
Description | Data deposited in or computed by PubChem | |
Molecular Formula |
C9H20N2O2 | |
Source | PubChem | |
URL | https://pubchem.ncbi.nlm.nih.gov | |
Description | Data deposited in or computed by PubChem | |
DSSTOX Substance ID |
DTXSID20944351 | |
Record name | 7,8-Diaminononanoic acid | |
Source | EPA DSSTox | |
URL | https://comptox.epa.gov/dashboard/DTXSID20944351 | |
Description | DSSTox provides a high quality public chemistry resource for supporting improved predictive toxicology. | |
Molecular Weight |
188.27 g/mol | |
Source | PubChem | |
URL | https://pubchem.ncbi.nlm.nih.gov | |
Description | Data deposited in or computed by PubChem | |
CAS No. |
21738-21-6 | |
Record name | 7,8-Diaminononanoic acid | |
Source | CAS Common Chemistry | |
URL | https://commonchemistry.cas.org/detail?cas_rn=21738-21-6 | |
Description | CAS Common Chemistry is an open community resource for accessing chemical information. Nearly 500,000 chemical substances from CAS REGISTRY cover areas of community interest, including common and frequently regulated chemicals, and those relevant to high school and undergraduate chemistry classes. This chemical information, curated by our expert scientists, is provided in alignment with our mission as a division of the American Chemical Society. | |
Explanation | The data from CAS Common Chemistry is provided under a CC-BY-NC 4.0 license, unless otherwise stated. | |
Record name | 7,8-Diaminopelargonic acid | |
Source | ChemIDplus | |
URL | https://pubchem.ncbi.nlm.nih.gov/substance/?source=chemidplus&sourceid=0021738216 | |
Description | ChemIDplus is a free, web search system that provides access to the structure and nomenclature authority files used for the identification of chemical substances cited in National Library of Medicine (NLM) databases, including the TOXNET system. | |
Record name | 7,8-Diaminononanoic acid | |
Source | EPA DSSTox | |
URL | https://comptox.epa.gov/dashboard/DTXSID20944351 | |
Description | DSSTox provides a high quality public chemistry resource for supporting improved predictive toxicology. | |
Retrosynthesis Analysis
AI-Powered Synthesis Planning: Our tool employs the Template_relevance Pistachio, Template_relevance Bkms_metabolic, Template_relevance Pistachio_ringbreaker, Template_relevance Reaxys, Template_relevance Reaxys_biocatalysis model, leveraging a vast database of chemical reactions to predict feasible synthetic routes.
One-Step Synthesis Focus: Specifically designed for one-step synthesis, it provides concise and direct routes for your target compounds, streamlining the synthesis process.
Accurate Predictions: Utilizing the extensive PISTACHIO, BKMS_METABOLIC, PISTACHIO_RINGBREAKER, REAXYS, REAXYS_BIOCATALYSIS database, our tool offers high-accuracy predictions, reflecting the latest in chemical research and data.
Strategy Settings
Precursor scoring | Relevance Heuristic |
---|---|
Min. plausibility | 0.01 |
Model | Template_relevance |
Template Set | Pistachio/Bkms_metabolic/Pistachio_ringbreaker/Reaxys/Reaxys_biocatalysis |
Top-N result to add to graph | 6 |
Feasible Synthetic Routes
Disclaimer and Information on In-Vitro Research Products
Please be aware that all articles and product information presented on BenchChem are intended solely for informational purposes. The products available for purchase on BenchChem are specifically designed for in-vitro studies, which are conducted outside of living organisms. In-vitro studies, derived from the Latin term "in glass," involve experiments performed in controlled laboratory settings using cells or tissues. It is important to note that these products are not categorized as medicines or drugs, and they have not received approval from the FDA for the prevention, treatment, or cure of any medical condition, ailment, or disease. We must emphasize that any form of bodily introduction of these products into humans or animals is strictly prohibited by law. It is essential to adhere to these guidelines to ensure compliance with legal and ethical standards in research and experimentation.