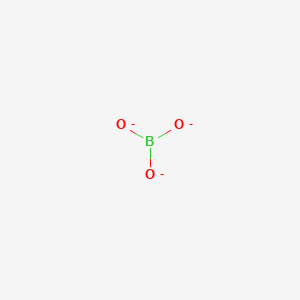
Borate
Overview
Description
Borate is a borate ion. It is a conjugate base of a hydrogenborate.
Scientific Research Applications
Borate Glasses: Unique Properties and Industrial Applications
Borate glasses, initially a scientific curiosity, have evolved to offer unique properties and applications in science and industry. Despite the dominance of silicate and borosilicate glasses in most areas, borate glasses have distinct advantages in certain scenarios. Their structure and affinity to water necessitate careful selection and development for specific applications. This review sheds light on the key properties of binary borate glasses and their potential industrial uses (Bengisu, 2016).
Lithium Borate Glass: Dosimetric Features and Transition Metal Doping
Lithium borate glasses doped with transition metals like Dysprosium have been studied for their dosimetric features. These glasses exhibit certain advantages over silicate glasses and are particularly significant in scientific and industrial applications where borate glasses' unique behavior, especially their interaction with water, is a consideration (Godwin et al., 2023).
Borate in Nonlinear Optical Materials
Borate-based materials are instrumental in producing deep-ultraviolet nonlinear optical materials. Their noncentrosymmetric structures, wide optical transparency, and large polarizabilities make them ideal for applications like photolithography and photochemical synthesis. The research provides strategies to enhance the design and discovery of these materials, including the introduction of fluorine atoms to improve optical properties (Mutailipu et al., 2019).
Optical Materials from Borates
Borates are a significant source of optical materials due to their complex structures and chemical properties. More than 3900 boron-containing compounds have been documented, offering potential for advances in nonlinear optical, birefringent, and self-frequency-doubling materials. The relationship between their structural architectures and optical properties is emphasized, highlighting the importance of borates in developing advanced optical materials (Mutailipu et al., 2020).
Multifunctional Lead Borate Whiskers
Lead borate, synthesized via a solvothermal method, has shown promising multifunctional applications. This material displays significant properties in attenuating γ-rays and neutrons, and exhibits good photoluminescence properties. This study indicates the potential for new applications in integrated multifunction whiskers (Sun et al., 2016).
properties
CAS RN |
14213-97-9 |
---|---|
Product Name |
Borate |
Molecular Formula |
BO3-3 |
Molecular Weight |
58.81 g/mol |
IUPAC Name |
borate |
InChI |
InChI=1S/BO3/c2-1(3)4/q-3 |
InChI Key |
BTBUEUYNUDRHOZ-UHFFFAOYSA-N |
SMILES |
B([O-])([O-])[O-] |
Canonical SMILES |
B([O-])([O-])[O-] |
Origin of Product |
United States |
Synthesis routes and methods I
Procedure details
Synthesis routes and methods II
Procedure details
Disclaimer and Information on In-Vitro Research Products
Please be aware that all articles and product information presented on BenchChem are intended solely for informational purposes. The products available for purchase on BenchChem are specifically designed for in-vitro studies, which are conducted outside of living organisms. In-vitro studies, derived from the Latin term "in glass," involve experiments performed in controlled laboratory settings using cells or tissues. It is important to note that these products are not categorized as medicines or drugs, and they have not received approval from the FDA for the prevention, treatment, or cure of any medical condition, ailment, or disease. We must emphasize that any form of bodily introduction of these products into humans or animals is strictly prohibited by law. It is essential to adhere to these guidelines to ensure compliance with legal and ethical standards in research and experimentation.