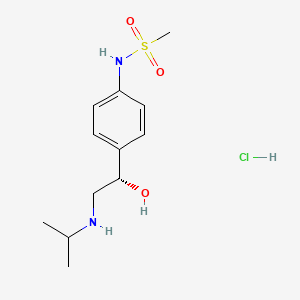
Dexsotalol hydrochloride
- Click on QUICK INQUIRY to receive a quote from our team of experts.
- With the quality product at a COMPETITIVE price, you can focus more on your research.
Overview
Description
Dexsotalol hydrochloride is a hydrochloride salt that is the monohydrochloride of sotalol. It has both beta-adrenoreceptor blocking (Vaughan Williams Class II) and cardiac action potential duration prolongation (Vaughan Williams Class III) antiarrhythmic properties . This compound is primarily used in the management of ventricular and supraventricular arrhythmias .
Preparation Methods
Synthetic Routes and Reaction Conditions
Dexsotalol hydrochloride can be synthesized through the reaction of sotalol with hydrochloric acid. The process involves the formation of a hydrochloride salt from the base compound, sotalol . The reaction conditions typically include the use of an aqueous solution of hydrochloric acid and sotalol, followed by crystallization to obtain the hydrochloride salt.
Industrial Production Methods
In industrial settings, the production of this compound involves large-scale synthesis using similar reaction conditions as in laboratory synthesis. The process is optimized for yield and purity, often involving multiple steps of purification and crystallization to ensure the final product meets pharmaceutical standards .
Chemical Reactions Analysis
Types of Reactions
Dexsotalol hydrochloride undergoes various chemical reactions, including:
Oxidation: This reaction involves the addition of oxygen or the removal of hydrogen from the compound.
Reduction: This reaction involves the addition of hydrogen or the removal of oxygen from the compound.
Substitution: This reaction involves the replacement of one atom or group of atoms in the compound with another atom or group of atoms.
Common Reagents and Conditions
Common reagents used in these reactions include oxidizing agents like potassium permanganate, reducing agents like lithium aluminum hydride, and various nucleophiles for substitution reactions. The conditions for these reactions vary depending on the desired product and the specific reaction being carried out .
Major Products Formed
The major products formed from these reactions depend on the specific type of reaction and the reagents used. For example, oxidation of this compound may yield oxidized derivatives, while reduction may produce reduced forms of the compound .
Scientific Research Applications
Dexsotalol hydrochloride has a wide range of scientific research applications, including:
Chemistry: Used as a reagent in various chemical reactions and studies.
Biology: Studied for its effects on biological systems, particularly in relation to its beta-adrenoreceptor blocking properties.
Medicine: Used in the treatment of arrhythmias and studied for its potential in other therapeutic areas.
Industry: Utilized in the production of pharmaceuticals and other chemical products
Mechanism of Action
Dexsotalol hydrochloride exerts its effects through its beta-adrenoreceptor blocking and cardiac action potential duration prolongation properties. It acts as a competitive inhibitor of the rapid potassium channel, which lengthens the duration of action potentials and the refractory period in the atria and ventricles . This inhibition is more pronounced at lower heart rates, which can lead to adverse effects like torsades de pointes . Additionally, the beta-blocking activity of this compound further prolongs action potentials and reduces the strength of contractility of muscle cells in the heart .
Comparison with Similar Compounds
Dexsotalol hydrochloride is similar to other beta-blockers and antiarrhythmic agents, such as:
Metoprolol: A selective beta-blocker used to lower blood pressure and relieve angina symptoms.
Xarelto (rivaroxaban): An anticoagulant used to prevent blood clots.
Uniqueness
What sets this compound apart is its dual action as both a beta-adrenoreceptor blocker and a cardiac action potential duration prolongation agent. This combination of properties makes it particularly effective in managing both ventricular and supraventricular arrhythmias .
List of Similar Compounds
- Metoprolol
- Xarelto (rivaroxaban)
- Other beta-blockers and antiarrhythmic agents
Properties
CAS No. |
4549-94-4 |
---|---|
Molecular Formula |
C12H21ClN2O3S |
Molecular Weight |
308.83 g/mol |
IUPAC Name |
N-[4-[(1S)-1-hydroxy-2-(propan-2-ylamino)ethyl]phenyl]methanesulfonamide;hydrochloride |
InChI |
InChI=1S/C12H20N2O3S.ClH/c1-9(2)13-8-12(15)10-4-6-11(7-5-10)14-18(3,16)17;/h4-7,9,12-15H,8H2,1-3H3;1H/t12-;/m1./s1 |
InChI Key |
VIDRYROWYFWGSY-UTONKHPSSA-N |
Isomeric SMILES |
CC(C)NC[C@H](C1=CC=C(C=C1)NS(=O)(=O)C)O.Cl |
SMILES |
CC(C)NCC(C1=CC=C(C=C1)NS(=O)(=O)C)O.Cl |
Canonical SMILES |
CC(C)NCC(C1=CC=C(C=C1)NS(=O)(=O)C)O.Cl |
4549-94-4 | |
Origin of Product |
United States |
Disclaimer and Information on In-Vitro Research Products
Please be aware that all articles and product information presented on BenchChem are intended solely for informational purposes. The products available for purchase on BenchChem are specifically designed for in-vitro studies, which are conducted outside of living organisms. In-vitro studies, derived from the Latin term "in glass," involve experiments performed in controlled laboratory settings using cells or tissues. It is important to note that these products are not categorized as medicines or drugs, and they have not received approval from the FDA for the prevention, treatment, or cure of any medical condition, ailment, or disease. We must emphasize that any form of bodily introduction of these products into humans or animals is strictly prohibited by law. It is essential to adhere to these guidelines to ensure compliance with legal and ethical standards in research and experimentation.