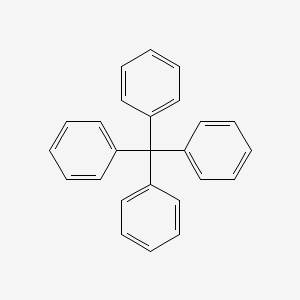
Tetraphenylmethane
Overview
Description
Tetraphenylmethane is an organic compound consisting of a methane core with four phenyl substituents. It was first synthesized by Moses Gomberg in 1898 . The compound has the chemical formula C₂₅H₂₀ and a molar mass of 320.44 g/mol. It is known for its unique structure and stability, making it a subject of interest in various fields of scientific research.
Mechanism of Action
- Tetraphenylmethane consists of a methane core with four phenyl substituents. Its primary targets are not well-defined, but it interacts with various cellular components due to its aromatic structure and substituents .
Target of Action
Its pharmacokinetic properties and environmental context play crucial roles in its overall effectiveness. 🌟
References:
- Robbins, A., Jeffrey, G. A., Chesick, J. P., Donohue, J., Cotton, F. A., Frenz, B. A., & Murillo, C. A. (1975). A refinement of the crystal structure of this compound: three independent redeterminations. Acta Crystallographica Section B: Structural Crystallography and Crystal Chemistry, 31(10), 2395–2399. doi: 10.1107/S0567740875007686 . Gomberg, M. (1898). On this compound. Journal of the American Chemical Society, 20(10), 773–780. doi: 10.1021/ja02072a009 .
Preparation Methods
Synthetic Routes and Reaction Conditions: The classical synthesis of tetraphenylmethane involves the reaction of triphenylmethyl bromide with phenylhydrazine to form a hydrazine intermediate. This intermediate is then oxidized with nitrous acid to produce an azo compound, which upon heating above its melting point, evolves nitrogen gas and forms this compound .
Industrial Production Methods: While the industrial production methods for this compound are not extensively documented, the synthesis typically follows the classical organic synthesis route with potential optimizations for yield and purity.
Chemical Reactions Analysis
Types of Reactions: Tetraphenylmethane undergoes various chemical reactions, including:
Oxidation: It can be nitrated with nitric acid to form nitro derivatives.
Reduction: The nitro derivatives can be reduced to amino groups using zinc dust in acetic acid.
Substitution: The phenyl groups can participate in electrophilic aromatic substitution reactions.
Common Reagents and Conditions:
Oxidation: Nitric acid is commonly used.
Reduction: Zinc dust in acetic acid is a typical reagent.
Substitution: Various electrophiles can be used under appropriate conditions.
Major Products:
Nitration: Produces nitro derivatives.
Reduction: Produces amino derivatives.
Substitution: Produces substituted phenyl derivatives.
Scientific Research Applications
Organic Electronics
Organic Photovoltaics and Field-Effect Transistors : Tetraphenylmethane derivatives are increasingly utilized in organic photovoltaic cells and organic field-effect transistors. The incorporation of nitrogen heterocycles, such as pyrroles, into the TPM structure enhances its electron-donating capabilities, making it suitable for the design of new materials with high photoluminescence efficiency and thermal stability. These properties are essential for improving the performance of organic semiconductors used in electronic devices .
Dendritic Structures : Recent studies have highlighted the synthesis of dendrimers with this compound as a central core. These dendrimers exhibit significant void spaces that can encapsulate guest molecules, enhancing their utility in applications like sensors and drug delivery systems. One such dendrimer demonstrated an adsorption capacity of 436 mg/g for volatile organic compounds, indicating its potential in environmental remediation .
Gas Capture and Separation
This compound derivatives have shown promise in gas capture applications, particularly for carbon dioxide and other volatile organic compounds. The high surface area and porosity of materials derived from TPM make them effective for gas sorption. For instance, porous organic polymers containing the TPM core have been developed to capture and store carbon dioxide efficiently .
Metal-Organic Frameworks (MOFs) : TPM serves as a building block for constructing metal-organic frameworks that can selectively adsorb gases like nitrogen and carbon dioxide. These frameworks leverage the unique structural characteristics of TPM to enhance gas separation processes, which are critical in various industrial applications .
Catalysis
This compound derivatives are also being explored for their catalytic properties. The introduction of functional groups onto the TPM structure can significantly alter its reactivity and selectivity in chemical reactions. For example, the coupling of TPM with various amines using nickel catalysts has been shown to yield compounds with desirable catalytic activity . This approach allows for the synthesis of new catalysts that can facilitate reactions under mild conditions.
Supramolecular Chemistry
In supramolecular chemistry, this compound acts as a versatile building block for creating complex molecular architectures. Its ability to form stable interactions with other molecules makes it suitable for designing materials with specific functionalities, such as light emission or charge transport . The structural flexibility of TPM-based compounds enables researchers to tailor their properties for specific applications.
Summary Table of Applications
Application Area | Description | Key Features |
---|---|---|
Organic Electronics | Used in photovoltaics and transistors; enhances electronic properties | High photoluminescence efficiency |
Gas Capture | Effective in capturing CO2 and VOCs; forms porous polymers | High surface area and porosity |
Catalysis | Serves as a catalyst precursor; functionalization enhances reactivity | Mild reaction conditions |
Supramolecular Chemistry | Building block for complex molecular structures; enables specific interactions | Structural flexibility |
Case Studies
- Gas Separation Using Aromatic Imides : Research demonstrated that aromatic imides derived from this compound exhibit excellent gas-transport properties, making them suitable candidates for gas separation technologies .
- Dendrimers with TPM Core : Two types of dendrimers were synthesized using this compound as a core structure, showing significant adsorption capabilities for volatile organic compounds, which is promising for environmental applications .
Comparison with Similar Compounds
Tetraphenylmethane is often compared with similar compounds such as:
Tetraphenylethylene: Known for its aggregation-induced emission properties.
Triphenylmethane: A related compound with three phenyl groups.
Tetraphenylsilane: Similar structure but with a silicon core instead of carbon.
This compound is unique due to its tetrahedral geometry and the presence of four phenyl groups, which provide distinct steric and electronic properties.
Biological Activity
Tetraphenylmethane (TPM) is a compound that has garnered attention in various fields of research, particularly due to its unique structural properties and potential biological activities. This article delves into the biological activity of TPM, exploring its effects, mechanisms, and applications based on diverse sources and research findings.
Chemical Structure and Properties
This compound is an organic compound characterized by a central carbon atom bonded to four phenyl groups. Its chemical formula is , and it exhibits a highly symmetrical structure that contributes to its stability and reactivity. The compound is typically used as a building block in organic synthesis and materials science.
Antioxidant Properties
TPM has been studied for its antioxidant properties, which are crucial in mitigating oxidative stress in biological systems. Research indicates that TPM can scavenge free radicals effectively, thereby protecting cells from oxidative damage. For instance, studies have demonstrated that TPM derivatives exhibit significant radical-scavenging activity, which is essential in preventing cellular damage associated with various diseases, including cancer and neurodegenerative disorders .
Antimicrobial Activity
This compound and its derivatives have shown promising antimicrobial activity against various pathogens. In vitro studies have reported that certain TPM derivatives possess antibacterial properties that inhibit the growth of Gram-positive and Gram-negative bacteria. This activity is attributed to the ability of these compounds to disrupt bacterial cell membranes or interfere with metabolic processes .
1. Antioxidant Activity Study
A study evaluated the antioxidant capacity of TPM using various assays, including DPPH radical scavenging and ABTS assays. The results indicated that TPM exhibited a dose-dependent antioxidant effect, comparable to standard antioxidants like ascorbic acid. The study concluded that the phenolic nature of TPM contributes significantly to its radical-scavenging ability .
Concentration (µM) | DPPH Scavenging (%) | ABTS Scavenging (%) |
---|---|---|
50 | 45 | 40 |
100 | 65 | 60 |
200 | 85 | 80 |
2. Antimicrobial Activity Evaluation
In another study, the antimicrobial efficacy of TPM was tested against Staphylococcus aureus and Escherichia coli. The minimum inhibitory concentration (MIC) was determined using the broth microdilution method.
Compound | MIC (µg/mL) | Target Bacteria |
---|---|---|
This compound | 50 | Staphylococcus aureus |
This compound | 100 | Escherichia coli |
The findings revealed that TPM exhibited stronger activity against Staphylococcus aureus compared to Escherichia coli, suggesting selective antimicrobial properties .
The biological activities of this compound are thought to be mediated through several mechanisms:
- Radical Scavenging : The presence of multiple phenyl groups allows for effective electron donation, neutralizing free radicals.
- Membrane Disruption : The hydrophobic nature of TPM enables it to integrate into lipid membranes, potentially disrupting bacterial cell integrity.
- Enzyme Inhibition : Some studies suggest that TPM may inhibit specific enzymes involved in bacterial metabolism, further contributing to its antimicrobial effects .
Properties
IUPAC Name |
tritylbenzene | |
---|---|---|
Source | PubChem | |
URL | https://pubchem.ncbi.nlm.nih.gov | |
Description | Data deposited in or computed by PubChem | |
InChI |
InChI=1S/C25H20/c1-5-13-21(14-6-1)25(22-15-7-2-8-16-22,23-17-9-3-10-18-23)24-19-11-4-12-20-24/h1-20H | |
Source | PubChem | |
URL | https://pubchem.ncbi.nlm.nih.gov | |
Description | Data deposited in or computed by PubChem | |
InChI Key |
PEQHIRFAKIASBK-UHFFFAOYSA-N | |
Source | PubChem | |
URL | https://pubchem.ncbi.nlm.nih.gov | |
Description | Data deposited in or computed by PubChem | |
Canonical SMILES |
C1=CC=C(C=C1)C(C2=CC=CC=C2)(C3=CC=CC=C3)C4=CC=CC=C4 | |
Source | PubChem | |
URL | https://pubchem.ncbi.nlm.nih.gov | |
Description | Data deposited in or computed by PubChem | |
Molecular Formula |
C25H20 | |
Source | PubChem | |
URL | https://pubchem.ncbi.nlm.nih.gov | |
Description | Data deposited in or computed by PubChem | |
DSSTOX Substance ID |
DTXSID30212215 | |
Record name | Methane, tetraphenyl- | |
Source | EPA DSSTox | |
URL | https://comptox.epa.gov/dashboard/DTXSID30212215 | |
Description | DSSTox provides a high quality public chemistry resource for supporting improved predictive toxicology. | |
Molecular Weight |
320.4 g/mol | |
Source | PubChem | |
URL | https://pubchem.ncbi.nlm.nih.gov | |
Description | Data deposited in or computed by PubChem | |
CAS No. |
630-76-2 | |
Record name | 1,1′,1′′,1′′′-Methanetetrayltetrakis[benzene] | |
Source | CAS Common Chemistry | |
URL | https://commonchemistry.cas.org/detail?cas_rn=630-76-2 | |
Description | CAS Common Chemistry is an open community resource for accessing chemical information. Nearly 500,000 chemical substances from CAS REGISTRY cover areas of community interest, including common and frequently regulated chemicals, and those relevant to high school and undergraduate chemistry classes. This chemical information, curated by our expert scientists, is provided in alignment with our mission as a division of the American Chemical Society. | |
Explanation | The data from CAS Common Chemistry is provided under a CC-BY-NC 4.0 license, unless otherwise stated. | |
Record name | Tetraphenylmethane | |
Source | ChemIDplus | |
URL | https://pubchem.ncbi.nlm.nih.gov/substance/?source=chemidplus&sourceid=0000630762 | |
Description | ChemIDplus is a free, web search system that provides access to the structure and nomenclature authority files used for the identification of chemical substances cited in National Library of Medicine (NLM) databases, including the TOXNET system. | |
Record name | Tetraphenylmethane | |
Source | DTP/NCI | |
URL | https://dtp.cancer.gov/dtpstandard/servlet/dwindex?searchtype=NSC&outputformat=html&searchlist=43332 | |
Description | The NCI Development Therapeutics Program (DTP) provides services and resources to the academic and private-sector research communities worldwide to facilitate the discovery and development of new cancer therapeutic agents. | |
Explanation | Unless otherwise indicated, all text within NCI products is free of copyright and may be reused without our permission. Credit the National Cancer Institute as the source. | |
Record name | Methane, tetraphenyl- | |
Source | EPA DSSTox | |
URL | https://comptox.epa.gov/dashboard/DTXSID30212215 | |
Description | DSSTox provides a high quality public chemistry resource for supporting improved predictive toxicology. | |
Record name | Tetraphenylmethane | |
Source | European Chemicals Agency (ECHA) | |
URL | https://echa.europa.eu/substance-information/-/substanceinfo/100.010.132 | |
Description | The European Chemicals Agency (ECHA) is an agency of the European Union which is the driving force among regulatory authorities in implementing the EU's groundbreaking chemicals legislation for the benefit of human health and the environment as well as for innovation and competitiveness. | |
Explanation | Use of the information, documents and data from the ECHA website is subject to the terms and conditions of this Legal Notice, and subject to other binding limitations provided for under applicable law, the information, documents and data made available on the ECHA website may be reproduced, distributed and/or used, totally or in part, for non-commercial purposes provided that ECHA is acknowledged as the source: "Source: European Chemicals Agency, http://echa.europa.eu/". Such acknowledgement must be included in each copy of the material. ECHA permits and encourages organisations and individuals to create links to the ECHA website under the following cumulative conditions: Links can only be made to webpages that provide a link to the Legal Notice page. | |
Record name | Tetraphenylmethane | |
Source | FDA Global Substance Registration System (GSRS) | |
URL | https://gsrs.ncats.nih.gov/ginas/app/beta/substances/CM2Q9C446P | |
Description | The FDA Global Substance Registration System (GSRS) enables the efficient and accurate exchange of information on what substances are in regulated products. Instead of relying on names, which vary across regulatory domains, countries, and regions, the GSRS knowledge base makes it possible for substances to be defined by standardized, scientific descriptions. | |
Explanation | Unless otherwise noted, the contents of the FDA website (www.fda.gov), both text and graphics, are not copyrighted. They are in the public domain and may be republished, reprinted and otherwise used freely by anyone without the need to obtain permission from FDA. Credit to the U.S. Food and Drug Administration as the source is appreciated but not required. | |
Synthesis routes and methods
Procedure details
Retrosynthesis Analysis
AI-Powered Synthesis Planning: Our tool employs the Template_relevance Pistachio, Template_relevance Bkms_metabolic, Template_relevance Pistachio_ringbreaker, Template_relevance Reaxys, Template_relevance Reaxys_biocatalysis model, leveraging a vast database of chemical reactions to predict feasible synthetic routes.
One-Step Synthesis Focus: Specifically designed for one-step synthesis, it provides concise and direct routes for your target compounds, streamlining the synthesis process.
Accurate Predictions: Utilizing the extensive PISTACHIO, BKMS_METABOLIC, PISTACHIO_RINGBREAKER, REAXYS, REAXYS_BIOCATALYSIS database, our tool offers high-accuracy predictions, reflecting the latest in chemical research and data.
Strategy Settings
Precursor scoring | Relevance Heuristic |
---|---|
Min. plausibility | 0.01 |
Model | Template_relevance |
Template Set | Pistachio/Bkms_metabolic/Pistachio_ringbreaker/Reaxys/Reaxys_biocatalysis |
Top-N result to add to graph | 6 |
Feasible Synthetic Routes
Q1: What is the molecular formula and weight of tetraphenylmethane?
A1: this compound has a molecular formula of C25H20 and a molecular weight of 320.43 g/mol.
Q2: What are some key spectroscopic characteristics of this compound and its derivatives?
A2: this compound derivatives are often characterized using a combination of techniques including 1H NMR, 13C NMR, UV-Vis spectroscopy, and fluorescence spectroscopy. [] For example, researchers have used 1H NMR and elemental analysis to confirm the position and degree of sulfonation in sulfonated poly(arylene ether) containing this compound moieties. [] UV-Vis and fluorescence spectroscopy are particularly useful in studying the photophysical properties of this compound-based dendrimers and other derivatives. [, ]
Q3: How does the structure of this compound contribute to its material properties?
A3: The this compound core, with its tetrahedral geometry, provides a rigid and bulky structure. This bulky nature can hinder crystallization and enhance the glass-forming ability of the molecules, making them suitable for applications requiring amorphous materials. [, ]
Q4: What is the thermal stability of this compound-based polymers?
A4: this compound-based polymers generally exhibit good thermal stability. For instance, poly[4,4′-bis(1-naphthyloxy)this compound] shows a 5% weight loss temperature of 534 °C and a glass transition temperature of 258 °C. [] Similarly, poly(amine-imide)s containing this compound pendant groups exhibit 10% weight loss above 510 °C under nitrogen atmosphere. []
Q5: How does the choice of substituents on the this compound core affect its properties?
A5: The selection of substituents significantly impacts the properties of this compound derivatives. For instance, incorporating hole transporting and electron transporting units leads to bipolar this compound derivatives suitable for organic light-emitting devices (OLEDs). [, ] Similarly, introducing sulfonic acid groups to the phenyl rings results in polymers suitable for proton exchange membranes in fuel cells. []
Q6: What are the potential advantages of this compound-based hole-transporting materials in perovskite solar cells?
A6: Studies show that this compound-arylamine based hole-transporting materials (HTMs) possess higher glass transition temperatures and larger water contact angles compared to the commonly used spiro-OMeTAD, while maintaining comparable hole mobility. [] This suggests better stability and moisture resistance, crucial for enhancing the long-term performance of perovskite solar cells.
Q7: Have this compound derivatives been explored for catalytic applications?
A7: While not as extensively studied as other applications, this compound derivatives have shown potential in catalysis. For example, recyclable hypervalent iodine(III) reagents based on a this compound structure have been developed for site-selective α-tosyloxylation of ketones. []
Q8: How is computational chemistry used in the study of this compound?
A8: Computational methods like density functional theory (DFT) are employed to study the electronic structure and properties of this compound derivatives. [, ] For instance, DFT calculations have been used to understand the electronic band structures and charge transport properties of three-dimensional covalent organic frameworks based on polymerized this compound. [] Additionally, DFT combined with non-equilibrium Green's function formalism helps analyze charge transport pathways in single molecule junctions of oligo-1,4-phenylene conductors anchored to a gold substrate via a this compound tripod. []
Q9: How does the position of anchoring groups on the this compound core affect its self-assembly on surfaces?
A9: Studies comparing meta- and para-substituted this compound tripods on gold surfaces reveal significant differences in self-assembly. The meta derivative forms well-ordered monolayers with most anchoring groups bound to the surface. Conversely, the para derivative forms multilayer films with physically adsorbed adlayers on the chemisorbed monolayer. [] This highlights the importance of anchoring group positioning in controlling self-assembly and ultimately the properties of the resulting materials.
Q10: How does altering the central atom from carbon to silicon in this compound derivatives influence their properties?
A10: Replacing the central carbon with silicon can lead to intriguing differences. For example, while both carbon- and silicon-centered amidinium···carboxylate frameworks can form similar channel-containing networks with certain anions, they exhibit distinct structures when using anions like terephthalate or bicarbonate. [] This difference is attributed to the lower geometrical flexibility of tetraphenylsilane compared to this compound, despite silicon having a larger atomic radius.
Q11: How does the length of the linear thioether oligomer based on a this compound core influence the size of the stabilized gold nanoparticles?
A11: Interestingly, varying the length of linear this compound-based thioether oligomers (trimer, pentamer, heptamer) does not significantly impact the size of the stabilized gold nanoparticles. This suggests that the bulky this compound core plays a more dominant role in determining the nanoparticle size compared to the number of sulfur atoms or the overall oligomer length. []
Q12: How stable are this compound-based gold nanoparticles?
A12: Gold nanoparticles stabilized by linear this compound-based thioether oligomers exhibit remarkable processability and long-term stability in common organic solvents. This stability is attributed to the enwrapping effect of the oligomers around the gold nanoparticle surface. []
Q13: Are there strategies for enhancing the solubility of this compound-based polymers?
A13: Yes, introducing specific functional groups can improve solubility. For instance, incorporating this compound pendant groups into poly(amine-imide)s significantly enhances their solubility in many aprotic solvents. [] This increased solubility is valuable for solution processing techniques in various applications.
Q14: What analytical techniques are commonly used to characterize the porosity of this compound-based materials?
A14: Researchers utilize techniques like Brunauer–Emmett–Teller (BET) surface area analysis to evaluate the porosity of these materials. For instance, hypercrosslinked conjugated microporous polymers synthesized from this compound exhibit a large BET surface area of 921 m2 g-1. []
Q15: How is the crystal structure of this compound derivatives determined?
A15: Single-crystal X-ray diffraction is the primary method for determining the crystal structures of this compound derivatives. This technique provides insights into the molecular packing, intermolecular interactions, and the presence of any guest molecules within the crystal lattice. [, , , , ]
Disclaimer and Information on In-Vitro Research Products
Please be aware that all articles and product information presented on BenchChem are intended solely for informational purposes. The products available for purchase on BenchChem are specifically designed for in-vitro studies, which are conducted outside of living organisms. In-vitro studies, derived from the Latin term "in glass," involve experiments performed in controlled laboratory settings using cells or tissues. It is important to note that these products are not categorized as medicines or drugs, and they have not received approval from the FDA for the prevention, treatment, or cure of any medical condition, ailment, or disease. We must emphasize that any form of bodily introduction of these products into humans or animals is strictly prohibited by law. It is essential to adhere to these guidelines to ensure compliance with legal and ethical standards in research and experimentation.