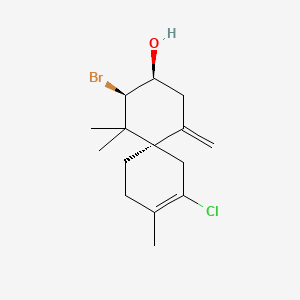
Elatol
Overview
Description
Elatol is a halogenated chamigrane-type sesquiterpene predominantly isolated from marine red algae of the genus Laurencia, notably Laurencia dendroidea and Laurencia microcladia. It is characterized by a bicyclic carbon skeleton with chlorine and bromine substitutions, contributing to its lipophilicity and bioactivity . This compound exhibits broad-spectrum biological activities, including antiparasitic, antileishmanial, antitumor, and antibacterial properties. Its mechanism of action often involves mitochondrial dysfunction, reactive oxygen species (ROS) overproduction, and inhibition of eukaryotic translation initiation factor 4A1 (eIF4A1), a key enzyme in cap-dependent protein synthesis .
Notably, this compound demonstrates selective toxicity against pathogens over mammalian cells. For example, it inhibits Leishmania amazonensis promastigotes and amastigotes with IC50 values of 4.0 µM and 0.45 µM, respectively, while showing minimal cytotoxicity to macrophages . Similarly, it exhibits potent activity against Trypanosoma cruzi (IC50 = 1.38 µM) and Naegleria fowleri (IC50 = 1.08 µM) .
Preparation Methods
Natural Extraction Methods from Laurencia Species
Elatol is predominantly obtained through extraction from marine red algae, particularly Laurencia dendroidea. Ecological studies have identified distinct chemotypes within Laurencia populations, with this compound constituting up to 50% of secondary metabolites in certain strains .
Solvent-Based Extraction Protocols
Fresh or dried Laurencia biomass is subjected to sequential solvent extraction. The standard protocol involves:
-
Washing : Algal thalli are rinsed with seawater to remove epiphytes and debris.
-
Drying : Biomass is air-dried at ambient temperature to preserve thermolabile compounds.
-
Extraction : Dried material is macerated in dichloromethane (DCM) for 72 hours, with three solvent renewals to maximize yield .
-
Concentration : The combined DCM phases are filtered and evaporated under reduced pressure using rotary evaporation.
This method yields a crude extract containing this compound alongside related sesquiterpenes like obtusol. Subsequent purification employs silica gel column chromatography with hexane/ethyl acetate gradients, achieving this compound purity >95% .
Chemotype-Specific Variability
Geographical distribution significantly impacts this compound content. For example:
-
Azeda Beach (Brazil) : Laurencia dendroidea populations yield this compound as the dominant metabolite (∼50% of extract) .
-
Forno Beach (Brazil) : Obtusol predominates, with this compound present only in trace amounts .
Such chemotypic variation necessitates population-specific extraction strategies to optimize this compound recovery.
Total Synthesis Approaches
The structural complexity of this compound, featuring a spiro[5.5]undecane core and vicinal quaternary stereocenters, has challenged synthetic chemists for decades. The first catalytic asymmetric total synthesis, reported by White et al., remains the benchmark .
Retrosynthetic Strategy
The synthesis dissects this compound into two key fragments:
-
Spirocyclic Core (A-ring) : Constructed via enantioselective decarboxylative allylation.
-
Chlorinated Olefin (B-ring) : Formed through ring-closing metathesis (RCM) and reductive transposition .
Enantioselective Decarboxylative Allylation
A palladium-catalyzed allylic alkylation installs the all-carbon quaternary stereocenter:
-
Substrate : Cyclohexenyl carboxylate.
-
Catalyst : Pd(0)/PHOX ligand system.
-
Conditions : Tetrahydrofuran (THF), -78°C, 12 hours.
Ring-Closing Metathesis
Grubbs 2nd-generation catalyst mediates spirocycle formation:
-
Substrate : Diethyl diallylmalonate derivative.
-
Catalyst : Ru-carbene complex (5 mol%).
-
Conditions : Dichloromethane, 40°C, 24 hours.
Reductive Olefin Transposition
A tandem hydroboration-oxidation sequence adjusts olefin positioning:
-
Hydroboration : 9-BBN in THF, 0°C to room temperature.
-
Oxidation : H₂O₂/NaOH, yielding secondary alcohol.
Diastereoselective Reduction
The final stereocenter is introduced via Evans-Przybylski reduction:
-
Substrate : α-Bromoketone intermediate.
-
Reagent : L-Selectride®.
-
Conditions : THF, -78°C, 2 hours.
Comparative Analysis of Preparation Methods
Parameter | Natural Extraction | Total Synthesis | Semi-Synthesis |
---|---|---|---|
Yield | 0.1–0.5% (dry weight) | 12% (overall) | 13–70% |
Purity | 95–98% | >99% | 85–95% |
Time Investment | 7–10 days | 15–20 steps | 1–3 steps |
Cost | Low (biomass) | High (catalysts) | Moderate |
Scalability | Limited by biomass | Multi-gram scale | Gram scale |
Key Observations :
-
Natural Extraction : Ecologically sustainable but constrained by algal availability and seasonal variability .
-
Total Synthesis : Enables large-scale production but requires expensive chiral catalysts and rigorous purification .
-
Semi-Synthesis : Balances efficiency and complexity, though dependent on extracted this compound .
Chemical Reactions Analysis
Types of Chemical Reactions
Elatol can undergo several types of chemical reactions:
-
Oxidation
-
Reduction
-
Substitution
Oxidation Reactions
This compound can be oxidized to form epoxides and other oxygenated derivatives.
Common Reagents and Conditions: Common oxidizing agents include hydrogen peroxide (H2O2) and m-chloroperbenzoic acid (m-CPBA).
Mechanism of Oxidation: Oxidation reactions involve the replacement of C-H bonds with C-X bonds, where X is a more electronegative element such as oxygen5. For example, alcohols can be oxidized to aldehydes or ketones, and aldehydes can be further oxidized to carboxylic acids .
Reduction Reactions
Reduction reactions can modify the double bonds and other functional groups within the this compound structure.
Common Reagents and Conditions: Reducing agents such as lithium aluminum hydride (LiAlH4) and sodium borohydride (NaBH4) are frequently used.
Mechanism of Reduction: Reduction typically corresponds to the addition of hydrogen (H2) across a double bond or a C-X bond, where X is a heteroatom5. This process increases the number of C-H bonds5.
Substitution Reactions
Halogen substitution reactions can introduce different halogen atoms into the this compound molecule.
Common Reagents and Conditions: Halogenation reactions often employ reagents like chlorine (Cl2) or bromine (Br2) under controlled conditions.
Mechanism of Substitution: Substitution involves replacing one atom or group of atoms with another. In the context of this compound, this could involve replacing a hydrogen atom with a halogen5.
Products
The major products formed from these reactions include various epoxides, reduced sesquiterpenes, and halogenated derivatives, each potentially exhibiting distinct biological activities.
This compound Derivatives and Cytotoxicity
Chemical modifications performed on this compound can result in derivatives with varying polarity, such as hemisuccinates, carbamates, and sulfamates. In vitro cytotoxicity evaluations against A459 and RD tumor cell lines have been performed with this compound derivatives, showing that structural modifications can lead to more active derivatives .
Biological Activity Mechanism
This compound exerts its biological effects primarily through the inhibition of protein translation by targeting the eukaryotic initiation factor 4A (eIF4A). By binding to eIF4A, this compound disrupts its helicase activity, leading to the inhibition of protein synthesis, which can induce apoptosis in cancer cells and trigger the integrated stress response . this compound binds to eIF4A1 in a 2:1 stoichiometry, disrupting helicase activity .
Scientific Research Applications
Chemistry: Elatol serves as a valuable model compound for studying halogenated sesquiterpenes and their synthetic pathways.
Biology: The compound exhibits significant antibacterial and antifungal activities, making it a potential candidate for developing new antimicrobial agents.
Medicine: this compound has shown promising cytotoxic effects against several cancer cell lines, including non-Hodgkin lymphomas and leukemia
Mechanism of Action
Elatol exerts its effects primarily through the inhibition of protein translation. It targets the eukaryotic initiation factor 4A (eIF4A), a key component of the cap-dependent translation initiation complex. By binding to eIF4A, this compound disrupts its helicase activity, leading to the inhibition of protein synthesis. This mechanism induces apoptosis in cancer cells and triggers the integrated stress response .
Comparison with Similar Compounds
Elatol belongs to a class of halogenated sesquiterpenes with structural and functional analogs. Below is a comparative analysis of this compound and related compounds:
Structural Analogues
Isoobtusol
- Structure : Shares a chamigrane skeleton but differs in hydroxyl group positioning.
- Bioactivity :
- Exhibits lower cytotoxicity than this compound against tumor cells (CC50 = 14.24 µM vs. This compound’s 6.24 µM in RD cells) .
- Shows weaker antiparasitic activity against Naegleria fowleri (IC50 >150 µM) compared to this compound (IC50 = 1.08 µM) .
- Antibacterial activity : Less potent than this compound against Klebsiella pneumoniae and Salmonella spp. .
(–)-Elatol (Enantiomer)
- Structure : Mirror-image stereochemistry of (+)-elatol.
- Bioactivity: 34-fold less potent against Naegleria fowleri (IC50 = 36.77 µM) . Induces mitochondrial depolarization in Trypanosoma cruzi but at higher concentrations than (+)-elatol .
Debromothis compound and Dechlorothis compound
- Structure : Lack bromine or chlorine atoms.
- Bioactivity :
Functional Analogues
Obtusol
- Structure : Chamigrane-type with differing halogenation (bromine at C-3).
- Bioactivity :
Rogiolol
- Structure : Oxygenated chamigrane derivative.
- Bioactivity :
Key Research Findings and Data Tables
Table 1. Antiparasitic Activity of this compound and Analogues
Table 2. Cytotoxicity of this compound and Derivatives
Compound | Cell Line | CC50 (µM) | Reference |
---|---|---|---|
This compound | RD (tumor) | 6.24 | |
This compound | L929 (fibroblast) | 1.0 | |
Isoobtusol | RD (tumor) | 14.24 | |
This compound semi-succinate | RD (tumor) | 4.93 |
Biological Activity
Elatol, a sesquiterpenoid derived from marine algae, particularly from species such as Laurencia microcladia and Laurencia dendroidea, has garnered significant attention due to its diverse biological activities. This article synthesizes findings from various studies to provide a comprehensive overview of this compound's biological activity, particularly its anticancer and antiparasitic properties.
This compound is characterized by its unique sesquiterpenoid structure, which contributes to its biological efficacy. Its mechanism of action primarily involves the inhibition of the eukaryotic translation initiation factor 4A1 (eIF4A1), a DEAD-box RNA helicase crucial for cap-dependent translation initiation. By inhibiting eIF4A1, this compound disrupts protein synthesis in cancer cells, leading to growth arrest and apoptosis.
Key Findings on Mechanism
- Inhibition of eIF4A1 : this compound exhibits specific inhibition of ATP hydrolysis by eIF4A1, impairing its helicase activity. This inhibition results in the loss of translationally regulated proteins in sensitive tumor cells .
- Mitochondrial Impact : this compound induces mitochondrial membrane depolarization and increases the production of reactive oxygen species (ROS), specifically superoxide anions, which are implicated in oxidative stress and apoptosis .
Anticancer Activity
This compound has demonstrated potent anticancer effects against various tumor types, particularly non-Hodgkin lymphomas and chronic lymphoid leukemia (CLL).
Study Overview
- Cell Lines Tested : In vitro studies utilized HEK293T and HeLa cell lines alongside leukemia and lymphoma cell lines.
- Mechanism : The compound triggers the OMA1-DELE1-ATF4 mitochondrial stress pathway, leading to apoptosis without affecting cytoplasmic protein synthesis .
- In Vivo Efficacy : this compound has shown significant activity against lymphoma xenografts in animal models, demonstrating its potential as a therapeutic agent .
Antiparasitic Activity
This compound also exhibits promising antiparasitic properties, particularly against Trypanosoma cruzi, the causative agent of Chagas disease.
Research Findings
- Trypanocidal Effects : Studies indicate that this compound effectively induces oxidative stress in T. cruzi amastigotes, leading to increased ROS production and subsequent cell death .
- Comparative Efficacy : The compound has been shown to have considerable trypanocidal activity at micromolar concentrations, highlighting its potential as a lead compound for developing new antiparasitic therapies .
Data Summary
Case Studies
- Cancer Treatment : In a study involving lymphoma cell lines, this compound was administered at varying concentrations, demonstrating significant cytotoxicity with minimal effects on normal cells. The study concluded that this compound could be a viable candidate for further clinical development against hematological malignancies .
- Antiparasitic Application : A phenotypic screen revealed that this compound effectively reduced viability in T. cruzi cultures, with flow cytometry confirming increased ROS levels post-treatment. These findings suggest this compound's potential as a novel treatment for Chagas disease .
Q & A
Basic Research Questions
Q. How can researchers design a reproducible experiment to investigate Elatol’s biological activity?
- Methodological Answer : Use the PICOT framework to structure the experiment:
- Population/Problem : Define the biological system (e.g., cell lines, animal models) and this compound’s target (e.g., antimicrobial, anticancer).
- Intervention : Specify this compound’s concentration range, solvent controls, and exposure duration.
- Comparison : Include positive/negative controls (e.g., standard drugs, solvent-only groups).
- Outcome : Quantify measurable endpoints (e.g., IC₅₀, inhibition zones, gene expression).
- Time : Define observation periods and sampling intervals.
- Ensure methods are detailed in the Materials and Methods section, including equipment models, chemical sources (e.g., supplier, purity), and statistical tests .
Q. What are the best practices for reporting this compound’s spectroscopic data (e.g., NMR, MS) to ensure reproducibility?
- Methodological Answer :
- NMR : Report solvent, frequency, reference peak (e.g., TMS), and integration values.
- Mass Spectrometry : Include ionization mode, resolution, and calibration standards.
- Supplementary Materials : Provide raw spectral data files and annotated spectra. For new compounds, include elemental analysis and purity validation (e.g., HPLC traces).
- Follow journal-specific guidelines for spectral presentation (e.g., peak labeling, axis scaling) .
Q. How should researchers validate this compound’s stability under experimental conditions (e.g., light, temperature)?
- Methodological Answer :
- Design accelerated stability studies : Expose this compound to stressors (UV light, 40–60°C, varying pH) and monitor degradation via HPLC or LC-MS.
- Use control samples stored in inert conditions (e.g., -80°C, dark).
- Report degradation products and quantify stability thresholds (e.g., ≥90% purity retained after 24 hours) .
Advanced Research Questions
Q. How can contradictory data on this compound’s mechanism of action be systematically analyzed?
- Methodological Answer :
-
Comparative Analysis : Tabulate conflicting findings (e.g., Table 1) and assess variables (dosage, assay type, model systems).
-
Statistical Reconciliation : Apply meta-analysis or sensitivity analysis to identify confounding factors (e.g., solvent interference, cell line heterogeneity).
-
Experimental Replication : Reproduce key studies with standardized protocols and orthogonal assays (e.g., combining enzymatic assays with molecular docking) .
Table 1 : Contradictory Reports on this compound’s Mechanism of Action
Study Model System Key Finding Proposed Mechanism Potential Confounders A (2023) HeLa cells Apoptosis induction Caspase-3 activation Serum-free conditions B (2024) MCF-7 cells Necrosis observed ROS overproduction High dosage (100 µM)
Q. What strategies are effective for integrating multidisciplinary data (e.g., omics, structural biology) to elucidate this compound’s bioactivity?
- Methodological Answer :
- Data Fusion : Use bioinformatics tools (e.g., STRING, KEGG) to overlay transcriptomic/proteomic data with this compound’s structural targets.
- Docking Studies : Validate hypotheses with molecular dynamics simulations (e.g., AutoDock Vina) and compare binding affinities across homologs.
- Cross-Validation : Correlate in vitro findings with in vivo pharmacokinetic data (e.g., bioavailability, metabolite profiling) .
Q. How can researchers address variability in this compound’s extraction yields from natural sources?
- Methodological Answer :
- Optimization Frameworks : Apply response surface methodology (RSM) to variables (solvent polarity, temperature, extraction time).
- Metabolomic Profiling : Use LC-MS/MS to identify co-extracted compounds affecting yield.
- Batch Consistency : Implement quality control protocols (e.g., HPLC fingerprinting for source authentication) .
Q. Data Management & Ethical Considerations
Q. What are the minimum data requirements for publishing this compound-related research to ensure transparency?
- Methodological Answer :
- Primary Data : Include raw spectra, chromatograms, and dose-response curves.
- Metadata : Document experimental conditions (temperature, humidity, equipment calibration dates).
- Repositories : Deposit large datasets in public repositories (e.g., Zenodo, Figshare) with persistent identifiers (DOIs) .
Q. How should researchers handle ethical concerns when using animal models to study this compound’s toxicity?
- Methodological Answer :
- Follow ARRIVE Guidelines : Report animal welfare metrics (e.g., pain/distress scoring, euthanasia methods).
- Ethics Review : Obtain approval from institutional committees and cite approval IDs in the manuscript.
- Reduction Strategies : Use in silico or in vitro models for preliminary screens to minimize animal use .
Properties
IUPAC Name |
(3S,4R,6R)-4-bromo-10-chloro-5,5,9-trimethyl-1-methylidenespiro[5.5]undec-9-en-3-ol | |
---|---|---|
Source | PubChem | |
URL | https://pubchem.ncbi.nlm.nih.gov | |
Description | Data deposited in or computed by PubChem | |
InChI |
InChI=1S/C15H22BrClO/c1-9-5-6-15(8-11(9)17)10(2)7-12(18)13(16)14(15,3)4/h12-13,18H,2,5-8H2,1,3-4H3/t12-,13-,15+/m0/s1 | |
Source | PubChem | |
URL | https://pubchem.ncbi.nlm.nih.gov | |
Description | Data deposited in or computed by PubChem | |
InChI Key |
HZKGRCIKQBHSNA-KCQAQPDRSA-N | |
Source | PubChem | |
URL | https://pubchem.ncbi.nlm.nih.gov | |
Description | Data deposited in or computed by PubChem | |
Canonical SMILES |
CC1=C(CC2(CC1)C(=C)CC(C(C2(C)C)Br)O)Cl | |
Source | PubChem | |
URL | https://pubchem.ncbi.nlm.nih.gov | |
Description | Data deposited in or computed by PubChem | |
Isomeric SMILES |
CC1=C(C[C@]2(CC1)C(=C)C[C@@H]([C@@H](C2(C)C)Br)O)Cl | |
Source | PubChem | |
URL | https://pubchem.ncbi.nlm.nih.gov | |
Description | Data deposited in or computed by PubChem | |
Molecular Formula |
C15H22BrClO | |
Source | PubChem | |
URL | https://pubchem.ncbi.nlm.nih.gov | |
Description | Data deposited in or computed by PubChem | |
Molecular Weight |
333.69 g/mol | |
Source | PubChem | |
URL | https://pubchem.ncbi.nlm.nih.gov | |
Description | Data deposited in or computed by PubChem | |
CAS No. |
55303-97-4 | |
Record name | Elatol | |
Source | CAS Common Chemistry | |
URL | https://commonchemistry.cas.org/detail?cas_rn=55303-97-4 | |
Description | CAS Common Chemistry is an open community resource for accessing chemical information. Nearly 500,000 chemical substances from CAS REGISTRY cover areas of community interest, including common and frequently regulated chemicals, and those relevant to high school and undergraduate chemistry classes. This chemical information, curated by our expert scientists, is provided in alignment with our mission as a division of the American Chemical Society. | |
Explanation | The data from CAS Common Chemistry is provided under a CC-BY-NC 4.0 license, unless otherwise stated. | |
Record name | Elatol | |
Source | ChemIDplus | |
URL | https://pubchem.ncbi.nlm.nih.gov/substance/?source=chemidplus&sourceid=0055303974 | |
Description | ChemIDplus is a free, web search system that provides access to the structure and nomenclature authority files used for the identification of chemical substances cited in National Library of Medicine (NLM) databases, including the TOXNET system. | |
Retrosynthesis Analysis
AI-Powered Synthesis Planning: Our tool employs the Template_relevance Pistachio, Template_relevance Bkms_metabolic, Template_relevance Pistachio_ringbreaker, Template_relevance Reaxys, Template_relevance Reaxys_biocatalysis model, leveraging a vast database of chemical reactions to predict feasible synthetic routes.
One-Step Synthesis Focus: Specifically designed for one-step synthesis, it provides concise and direct routes for your target compounds, streamlining the synthesis process.
Accurate Predictions: Utilizing the extensive PISTACHIO, BKMS_METABOLIC, PISTACHIO_RINGBREAKER, REAXYS, REAXYS_BIOCATALYSIS database, our tool offers high-accuracy predictions, reflecting the latest in chemical research and data.
Strategy Settings
Precursor scoring | Relevance Heuristic |
---|---|
Min. plausibility | 0.01 |
Model | Template_relevance |
Template Set | Pistachio/Bkms_metabolic/Pistachio_ringbreaker/Reaxys/Reaxys_biocatalysis |
Top-N result to add to graph | 6 |
Feasible Synthetic Routes
Disclaimer and Information on In-Vitro Research Products
Please be aware that all articles and product information presented on BenchChem are intended solely for informational purposes. The products available for purchase on BenchChem are specifically designed for in-vitro studies, which are conducted outside of living organisms. In-vitro studies, derived from the Latin term "in glass," involve experiments performed in controlled laboratory settings using cells or tissues. It is important to note that these products are not categorized as medicines or drugs, and they have not received approval from the FDA for the prevention, treatment, or cure of any medical condition, ailment, or disease. We must emphasize that any form of bodily introduction of these products into humans or animals is strictly prohibited by law. It is essential to adhere to these guidelines to ensure compliance with legal and ethical standards in research and experimentation.