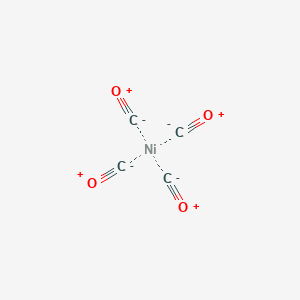
Nickel tetracarbonyl
Overview
Description
Nickel tetracarbonyl, also known as tetracarbonylnickel, is a nickel(0) organometallic compound with the chemical formula Ni(CO)₄. This colorless liquid is the principal carbonyl of nickel and is known for its high toxicity and volatility. It was first discovered by Ludwig Mond in 1890 and is primarily used in the Mond process for producing high-purity nickel .
Preparation Methods
Synthetic Routes and Reaction Conditions: Nickel tetracarbonyl is synthesized by the direct reaction of nickel metal with carbon monoxide. The reaction typically occurs at temperatures between 50°C and 60°C and under a pressure of 1-2 atmospheres of carbon monoxide:
Ni+4CO→Ni(CO)4
Industrial Production Methods: In industrial settings, this compound is produced through the Mond process. This process involves the following steps:
- Nickel oxide is reduced to nickel metal using hydrogen gas.
- The nickel metal is then reacted with carbon monoxide to form this compound.
- The this compound is decomposed at higher temperatures to yield high-purity nickel and carbon monoxide .
Types of Reactions:
- this compound can be oxidized by halogens such as chlorine to form nickel chloride and carbon monoxide:
Oxidation: Ni(CO)4+2Cl2→NiCl2+4CO
this compound can be reduced to nickel metal by heating:Reduction: Ni(CO)4→Ni+4CO
Substitution: this compound can undergo substitution reactions with alkyl and aryl halides to form carbonylated organic products.
Common Reagents and Conditions:
Oxidation: Halogens (e.g., chlorine) under ambient conditions.
Reduction: Heating in the absence of air.
Substitution: Alkyl and aryl halides under mild conditions.
Major Products Formed:
Oxidation: Nickel chloride and carbon monoxide.
Reduction: Nickel metal and carbon monoxide.
Substitution: Carbonylated organic products.
Scientific Research Applications
Nickel tetracarbonyl has several applications in scientific research and industry:
Mechanism of Action
Nickel tetracarbonyl exerts its effects through the coordination of carbon monoxide ligands to the nickel center. The carbon monoxide ligands donate electron density to the nickel atom, stabilizing the compound. The molecular targets and pathways involved include the interaction of this compound with other metal centers and organic molecules, leading to the formation of various nickel-containing compounds .
Comparison with Similar Compounds
Nickel tetracarbonyl is unique among metal carbonyls due to its high volatility and toxicity. Similar compounds include:
- Chromium hexacarbonyl (Cr(CO)₆)
- Molybdenum hexacarbonyl (Mo(CO)₆)
- Tungsten hexacarbonyl (W(CO)₆)
- Iron pentacarbonyl (Fe(CO)₅)
These compounds share similar properties, such as being metal carbonyls, but differ in their metal centers and the number of carbon monoxide ligands. This compound is distinct due to its use in the Mond process and its high volatility .
Properties
CAS No. |
13463-39-3 |
---|---|
Molecular Formula |
C4NiO4 |
Molecular Weight |
170.73 g/mol |
IUPAC Name |
carbon monoxide;nickel |
InChI |
InChI=1S/4CO.Ni/c4*1-2; |
InChI Key |
AWDHUGLHGCVIEG-UHFFFAOYSA-N |
SMILES |
[C-]#[O+].[C-]#[O+].[C-]#[O+].[C-]#[O+].[Ni] |
Canonical SMILES |
[C-]#[O+].[C-]#[O+].[C-]#[O+].[C-]#[O+].[Ni] |
boiling_point |
43 °C |
density |
Relative density (water = 1): 1.3 |
melting_point |
-25 °C |
13463-39-3 | |
physical_description |
VOLATILE COLOURLESS LIQUID WITH CHARACTERISTIC ODOUR. |
solubility |
Solubility in water, g/l at 20 °C: 0.02 (practically insoluble) |
Synonyms |
nickel carbonyl nickel tetracarbonyl |
vapor_density |
Relative vapor density (air = 1): 5.9 |
vapor_pressure |
Vapor pressure, kPa at 25.8 °C: 53 |
Origin of Product |
United States |
Synthesis routes and methods I
Procedure details
Synthesis routes and methods II
Procedure details
Disclaimer and Information on In-Vitro Research Products
Please be aware that all articles and product information presented on BenchChem are intended solely for informational purposes. The products available for purchase on BenchChem are specifically designed for in-vitro studies, which are conducted outside of living organisms. In-vitro studies, derived from the Latin term "in glass," involve experiments performed in controlled laboratory settings using cells or tissues. It is important to note that these products are not categorized as medicines or drugs, and they have not received approval from the FDA for the prevention, treatment, or cure of any medical condition, ailment, or disease. We must emphasize that any form of bodily introduction of these products into humans or animals is strictly prohibited by law. It is essential to adhere to these guidelines to ensure compliance with legal and ethical standards in research and experimentation.