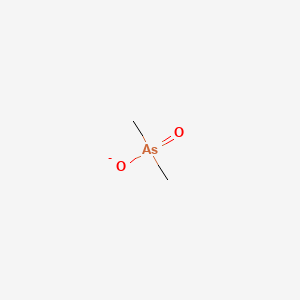
Dimethylarsinate
- Click on QUICK INQUIRY to receive a quote from our team of experts.
- With the quality product at a COMPETITIVE price, you can focus more on your research.
Overview
Description
Dimethylarsinate (DMA), also known as dimethylarsinic acid, is an organic arsenic compound formed during the metabolic detoxification of inorganic arsenic in humans and other organisms. After ingestion, inorganic arsenic (As³⁺ or As⁵⁺) undergoes methylation in the liver, producing monomethylarsonate (MMA) and subsequently DMA . DMA is less toxic than its inorganic precursors due to reduced cellular absorption and rapid urinary excretion, making it a key biomarker for arsenic exposure .
Preparation Methods
Synthetic Routes and Reaction Conditions: The cacodylate ion is typically prepared by neutralizing cacodylic acid with a base. The reaction can be represented as follows:
(CH3)2AsO2H+NaOH→(CH3)2AsO2Na+H2O
In this reaction, sodium hydroxide (NaOH) is used as the base to neutralize cacodylic acid, resulting in the formation of sodium cacodylate and water .
Industrial Production Methods: Industrial production of cacodylate ions involves the large-scale neutralization of cacodylic acid with sodium hydroxide. The process is carried out in controlled conditions to ensure the purity and stability of the final product .
Chemical Reactions Analysis
Degradation and Oxidation
-
Electro-Fenton Process Dimethylarsinate (DMA) can be efficiently oxidized into As(V) . In one study, 96% of DMA degraded after a 360-minute reaction time, resulting in residual As(V) concentration in the solution being below the allowable limit of 0.01 mg/L . The oxidation of DMA to MMA (monomethylarsonate) is the primary oxidation product, which is subsequently oxidized to inorganic arsenic, As(V) .
-
Role of Reactive Oxygen Species The reactive oxygen species, OH, is involved in the degradation process of DMA .
-
Transformation of Arsenic Species this compound, arsenocholine, and arsenobetaine can convert to As(V) during in vitro digestions, with varying efficiencies. The transformation is affected by pH, time, and digestion juice, with low pH being particularly important .
Use as a Catalyst
-
Catalysis of Chemical Reactions this compound can also act as a catalyst for independent chemical reactions without affecting its oscillating properties .
Other reactions
-
Reaction with Electrophiles: Dimethyl sulfoxide (DMSO) reacts violently with electrophiles like acetyl chloride, benzoyl chloride, and thionyl chloride . The byproducts include acids and/or chloride ions, which could further lower the onset temperature and increase the severity of DMSO decomposition, aggravating potential safety hazards .
-
Reaction with Reductants: DMSO can be reduced to dimethyl sulfide by certain reductants .
-
Reaction with Glyoxylic Acid: Glyoxylic acid can be added to catechol to form 3,4-dihydroxymandelic acid intermediate .
Scientific Research Applications
Agricultural Applications
Herbicide Use
Dimethylarsinate has historically been utilized as a non-selective herbicide. It is particularly noted for its effectiveness in cotton defoliation and weed control. The sodium salt of this compound is commonly used in agricultural practices to manage unwanted vegetation around crops and in non-crop areas. Its application includes:
- Cotton Defoliation : Used to promote leaf drop before harvesting.
- Weed Control : Effective in controlling various weed species around non-bearing citrus and other crops.
Environmental Applications
Degradation of this compound
Recent studies have highlighted the potential for using advanced oxidation processes to degrade this compound in contaminated environments. For instance, a study demonstrated the use of a FeCx/N-doped carbon fiber composite in an electro-Fenton process to efficiently convert this compound into less harmful inorganic arsenic (As(V)). Key findings include:
- Degradation Efficiency : Up to 96% degradation of this compound was achieved within 360 minutes.
- Mechanism : The process involved hydroxyl radicals as the primary reactive species for degradation, leading to the formation of methylarsinic acid before conversion to inorganic arsenic .
Medical Applications
Therapeutic Uses
this compound has been explored for its potential therapeutic applications, particularly in veterinary medicine. It has been used to treat conditions such as:
- Anemia : Administered as a treatment option for certain types of anemia in animals.
- Dermatological Conditions : Utilized for treating skin ailments due to its properties as a dermatologic agent.
Toxicological Studies
This compound is recognized for its toxicity, which has prompted extensive research into its health effects. Studies have shown that it can cause genotoxicity and teratogenic effects in animal models. Notably:
- Genotoxic Effects : Research indicates that this compound can lead to DNA damage and apoptosis in human cells, raising concerns about its safety .
- Environmental Impact : Investigations into the presence of this compound in food sources, particularly seafood, have linked it to increased urinary arsenic concentrations among consumers .
Analytical Applications
This compound is also significant in analytical chemistry, particularly for monitoring arsenic levels in biological and environmental samples. Techniques such as high-performance liquid chromatography coupled with mass spectrometry are employed to quantify this compound alongside other arsenic species .
Data Summary
Mechanism of Action
The primary function of the cacodylate ion in scientific applications is to act as a buffering agent. It stabilizes the pH of solutions by existing in equilibrium between its weak acid (cacodylic acid) and conjugate base (cacodylate ion) forms. When an acid is introduced, it reacts with the cacodylate ion to form cacodylic acid, minimizing changes in pH. Conversely, when a base is added, cacodylic acid donates a proton to neutralize the base, converting into the cacodylate ion .
Comparison with Similar Compounds
Comparison with Similar Arsenic Compounds
Metabolic Pathways and Excretion
DMA is distinguished from inorganic arsenic and other methylated species by its excretion kinetics. A seminal study by Buchet et al. (1981) compared urinary excretion profiles after single oral doses of sodium arsenite (As³⁺), MMA, and DMA in humans:
- Sodium arsenite : Metabolized into MMA (6–12% of dose) and DMA (2–4%) within 4 days .
- MMA : Further methylated to DMA, with 35–44% excreted as DMA .
Table 1: Excretion Profiles of Arsenic Compounds
Compound Administered | Major Metabolites Excreted | % Dose Excreted as DMA |
---|---|---|
Sodium arsenite | MMA, DMA | 2–4% |
MMA | DMA | 35–44% |
DMA | DMA | 65–75% |
Toxicity and Health Impacts
- Inorganic Arsenic (As³⁺/As⁵⁺): Strongly linked to cancers, cardiovascular diseases, and diabetes. Total arsenic levels in urine correlate with a 26% higher prevalence of type 2 diabetes .
- DMA: Considered less toxic than inorganic arsenic and MMA. However, elevated DMA levels show non-significant associations with diabetes (10% higher prevalence, 95% CI: -8.0% to 33.0%) .
Table 2: Health Outcomes of Arsenic Species
Environmental Behavior
- Soil Transformation: DMA in soil produces dimethylarsine gas, but at lower quantities than arsine from inorganic arsenate. For example, dimethylarsine yields from DMA are threefold lower than arsine from arsenate .
- Aquifer Cycling : DMA correlates with arsenite (As³⁺) in volcaniclastic aquifers, suggesting microbial methylation contributes to arsenic mobility .
Table 3: Environmental Transformation of Arsenic Species
Substrate | Gaseous Product | Relative Yield | Environmental Relevance |
---|---|---|---|
Arsenate (As⁵⁺) | Arsine | High | Major groundwater contaminant |
DMA | Dimethylarsine | Low | Minor contributor to volatilization |
Analytical Differentiation
DMA shares spectral similarities with other As(V) organoarsenicals (e.g., MMA, trimethylarsine oxide) in X-ray absorption near-edge structure (XANES) analysis. However, its distinct white-line peak energy (∼11,874 eV) and spectral shape allow differentiation from arsenobetaine and tetramethylarsonium .
Biological Activity
Dimethylarsinate (DMA) is a methylated metabolite of inorganic arsenic, primarily formed in the liver through the methylation process mediated by enzymes such as arsenic methyltransferase (AS3MT). Understanding its biological activity is crucial due to its implications in both toxicology and potential therapeutic applications. This article explores the biological activity of this compound, drawing on various studies and case reports.
1. Metabolism and Toxicity
This compound is produced when inorganic arsenic undergoes methylation in the body. This process involves the transfer of a methyl group from S-adenosylmethionine (SAM) to arsenic, resulting in the formation of DMA and other metabolites like monomethylarsonic acid (MMA) . The metabolism of arsenic compounds, including DMA, can have paradoxical effects; while some metabolites exhibit toxicity, others may possess anticancer properties.
Table 1: Methylation Pathway of Arsenic Compounds
Compound | Structure Type | Biological Activity |
---|---|---|
Inorganic Arsenic | iAs | Toxicity |
Monomethylarsonic Acid (MMA) | Methylated | Potential anticancer effects |
Dimethylarsinic Acid (DMA) | Methylated | Toxicity & possible therapeutic effects |
2.1 Toxicological Impacts
Studies indicate that exposure to this compound can lead to various health issues, including peripheral neuropathy and skin lesions. A case study reported a 35-year-old male with symptoms consistent with chronic arsenic exposure, highlighting the neurological and dermatological manifestations associated with high levels of arsenic metabolites in urine .
2.2 Anticancer Potential
Interestingly, some research suggests that this compound may play a role in cancer therapy. For instance, it has been shown that DMA and its metabolites can induce DNA hypomethylation, which is linked to the reactivation of tumor suppressor genes . Clinical studies involving acute promyelocytic leukemia (APL) patients demonstrated that methylated arsenic species were present in their blood after treatment, suggesting a potential therapeutic role for these metabolites .
3. Epidemiological Studies
Epidemiological data have linked urinary levels of this compound to various health outcomes. A study analyzing urine samples from U.S. adults found that those with higher levels of total arsenic and this compound had an increased risk of developing type 2 diabetes . This correlation emphasizes the need for further investigation into the long-term effects of DMA exposure on metabolic health.
Table 2: Urinary Levels of Arsenic Metabolites in Relation to Health Outcomes
Health Outcome | Total Arsenic (μg/L) | This compound (μg/L) | Odds Ratio |
---|---|---|---|
Type 2 Diabetes | 7.1 | 3.0 | 1.57 |
4. Microbial Interactions
Recent studies have explored how microbial communities interact with arsenic compounds, including this compound. Some microorganisms can utilize methylarsenicals as part of their metabolic processes, potentially influencing arsenic cycling in the environment . This microbial metabolism may also contribute to the toxicity profiles observed in higher trophic levels.
5. Conclusion
This compound exhibits complex biological activity characterized by both toxic and potentially therapeutic effects. Its metabolism and interaction with biological systems underscore the need for continued research into its implications for human health and disease management. Understanding these dynamics will be essential for developing effective strategies for mitigating arsenic-related health risks while exploring its therapeutic potential.
Q & A
Basic Research Questions
Q. What are the validated analytical methods for detecting dimethylarsinate in biological samples, and how do researchers ensure reproducibility?
- Methodological Answer: this compound is commonly quantified using high-performance liquid chromatography coupled with inductively coupled plasma mass spectrometry (HPLC-ICP-MS) due to its sensitivity for arsenic speciation. Urine samples are typically acidified to stabilize metabolites, followed by separation using anion-exchange columns. Method validation requires assessing limits of detection (LOD < 0.1 µg/L), recovery rates (85–110%), and cross-calibration with certified reference materials (e.g., NIST SRM 2669) . Reproducibility is ensured through inter-laboratory comparisons and adherence to protocols from large-scale studies like NHANES .
Q. How should researchers design a cross-sectional study to evaluate this compound exposure in human populations?
- Methodological Answer: Key steps include:
- Cohort Selection: Stratify participants by dietary habits (e.g., seafood consumption, a major source of organic arsenic) and demographics (age, sex) to control confounding factors .
- Sample Collection: Use spot urine samples adjusted for creatinine to account for hydration variability.
- Data Normalization: Apply multiple regression models to adjust for covariates like BMI and renal function .
- Ethical Compliance: Obtain informed consent and IRB approval, particularly for vulnerable populations .
Advanced Research Questions
Q. How can contradictory findings on this compound’s association with hyperuricemia be systematically resolved?
- Methodological Answer: Contradictions arise from variability in exposure sources (e.g., seafood vs. drinking water) and metabolic differences. Researchers should:
- Conduct sensitivity analyses to exclude outliers or high-leverage data points.
- Stratify analyses by exposure source using dietary questionnaires or arsenic speciation data .
- Perform meta-analyses of longitudinal datasets (e.g., NHANES, Korean intervention studies) to identify dose-response trends obscured in single studies .
- Validate findings with in vitro models (e.g., hepatic cell lines) to isolate this compound-specific effects from co-exposures .
Q. What experimental controls are critical when investigating this compound’s mechanisms in toxicological studies?
- Methodological Answer:
- Chemical Speciation Controls: Use arsenic standards (e.g., this compound vs. arsenobetaine) to distinguish organic vs. inorganic arsenic effects.
- Cell Culture Models: Include primary human hepatocytes or renal proximal tubule cells to replicate metabolic pathways relevant to humans.
- Exposure Duration: Short-term (acute) vs. long-term (chronic) exposures must be clearly defined, as this compound’s toxicity may vary temporally .
- Negative/Positive Controls: Include untreated controls and cells exposed to known nephrotoxicants (e.g., cisplatin) to benchmark results .
Q. How can researchers validate novel analytical methods for this compound in complex environmental matrices?
- Methodological Answer:
- Matrix-Specific Validation: Spike recovery tests in soil, water, and biological samples to assess interference from co-occurring metals (e.g., lead, cadmium).
- Quality Assurance: Participate in proficiency testing programs (e.g., QUASIMEME) and use isotope dilution techniques to improve accuracy .
- Data Reporting: Follow ISO/IEC 17025 guidelines for reporting uncertainties, including expanded uncertainty intervals and method robustness under varying pH/temperature conditions .
Q. Data Integration and Reporting
Q. What strategies are effective for integrating multi-omics data to study this compound’s metabolic impacts?
- Methodological Answer: Combine metabolomics (e.g., LC-MS) with transcriptomics (RNA-seq) to identify pathways like glutathione metabolism altered by this compound. Use bioinformatics tools (e.g., MetaboAnalyst, KEGG) for pathway enrichment analysis. Cross-reference findings with epidemiological data to prioritize clinically relevant biomarkers .
Properties
CAS No. |
15132-04-4 |
---|---|
Molecular Formula |
C2H6AsO2- |
Molecular Weight |
136.99 g/mol |
IUPAC Name |
dimethylarsinate |
InChI |
InChI=1S/C2H7AsO2/c1-3(2,4)5/h1-2H3,(H,4,5)/p-1 |
InChI Key |
OGGXGZAMXPVRFZ-UHFFFAOYSA-M |
SMILES |
C[As](=O)(C)[O-] |
Canonical SMILES |
C[As](=O)(C)[O-] |
Key on ui other cas no. |
15132-04-4 |
Synonyms |
Acid, Cacodylic Acid, Dimethylarsinic Cacodylate Cacodylic Acid Dimethylarsinate Dimethylarsinic Acid |
Origin of Product |
United States |
Disclaimer and Information on In-Vitro Research Products
Please be aware that all articles and product information presented on BenchChem are intended solely for informational purposes. The products available for purchase on BenchChem are specifically designed for in-vitro studies, which are conducted outside of living organisms. In-vitro studies, derived from the Latin term "in glass," involve experiments performed in controlled laboratory settings using cells or tissues. It is important to note that these products are not categorized as medicines or drugs, and they have not received approval from the FDA for the prevention, treatment, or cure of any medical condition, ailment, or disease. We must emphasize that any form of bodily introduction of these products into humans or animals is strictly prohibited by law. It is essential to adhere to these guidelines to ensure compliance with legal and ethical standards in research and experimentation.