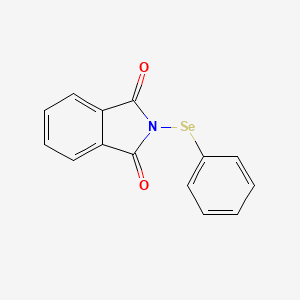
N-(Phenylseleno)phthalimide
Overview
Description
Mechanism of Action
Target of Action
N-(Phenylseleno)phthalimide is a versatile selenamide reagent . Its primary targets are thiol groups in proteins and peptides . Thiol groups, which contain sulfur, are found in cysteine residues and play crucial roles in protein structure and function .
Mode of Action
The compound interacts with its targets through a process known as derivatization . In this process, this compound selectively reacts with thiol groups, leading to the formation of a derivative that can be analyzed using mass spectrometry . This interaction is facilitated by the presence of adjacent basic amino acid residues, which stabilize the thiolate form, enhancing the nucleophilic attack on this compound .
Biochemical Pathways
The derivatization of thiol groups by this compound affects various biochemical pathways. For instance, it is used in α-selenenylation reactions of aldehydes and ketones . This reaction is promoted by L-prolinamide or pyrrolidine trifluoromethanesulfonamide
Result of Action
The primary result of this compound’s action is the selective derivatization of thiol groups . This allows for the detailed analysis of proteins and peptides containing these groups . For instance, it has been used to derivatize thiol peptides in protein digests . The selenium tags introduced by the compound can survive or be removed with collision-induced dissociation (CID), making them useful for mass spectrometric analysis .
Action Environment
The action of this compound can be influenced by various environmental factors. For example, the compound’s reactivity towards thiol groups reflects the chemical environment and accessibility of these groups in proteins and peptides . In the case of creatine phosphokinase, the native protein predominantly has one thiol group reacted with this compound, while all four thiol groups of the denatured protein can be derivatized . This suggests that the protein’s conformation can significantly influence the compound’s action.
Biochemical Analysis
Biochemical Properties
N-(Phenylseleno)phthalimide plays a crucial role in biochemical reactions by interacting with thiol groups in proteins and peptides. It is used to selectively derivatize thiols, which helps in the mass spectrometric analysis of these biomolecules . The compound reacts with thiol groups to form stable seleno derivatives, which can be easily detected and analyzed. This interaction is particularly useful in studying the structure and function of proteins and peptides, as it allows for the identification and quantification of thiol-containing biomolecules .
Cellular Effects
This compound has significant effects on various types of cells and cellular processes. It influences cell function by modifying thiol groups in proteins, which can affect cell signaling pathways, gene expression, and cellular metabolism . The compound’s ability to selectively derivatize thiols makes it a valuable tool for studying redox signaling and other cellular processes that involve thiol groups. By modifying thiol groups, this compound can alter the activity of enzymes and other proteins, leading to changes in cellular function and metabolism .
Molecular Mechanism
The molecular mechanism of this compound involves its interaction with thiol groups in proteins and peptides. The compound reacts with thiol groups to form stable seleno derivatives, which can be detected and analyzed using mass spectrometry . This reaction is facilitated by the nucleophilic attack of the thiol group on the selenium atom in this compound, resulting in the formation of a covalent bond between the thiol group and the selenium atom . This modification can affect the activity of enzymes and other proteins, leading to changes in cellular function and metabolism .
Temporal Effects in Laboratory Settings
In laboratory settings, the effects of this compound can change over time. The compound is stable under standard laboratory conditions, but its reactivity can be influenced by factors such as temperature, pH, and the presence of other chemicals . Over time, this compound may degrade, leading to changes in its effectiveness and the nature of its interactions with thiol groups . Long-term studies have shown that the compound can have lasting effects on cellular function, particularly in terms of redox signaling and other processes involving thiol groups .
Dosage Effects in Animal Models
The effects of this compound vary with different dosages in animal models. At low doses, the compound can selectively modify thiol groups without causing significant toxicity . At higher doses, this compound can have toxic effects, including damage to cellular structures and disruption of cellular function . Studies have shown that there is a threshold dose above which the compound’s toxic effects become more pronounced, leading to adverse effects on animal health .
Metabolic Pathways
This compound is involved in metabolic pathways that include the modification of thiol groups in proteins and peptides . The compound interacts with enzymes and cofactors that are involved in redox signaling and other cellular processes . By modifying thiol groups, this compound can affect metabolic flux and the levels of various metabolites . This makes it a valuable tool for studying the regulation of metabolic pathways and the role of thiol groups in cellular metabolism .
Transport and Distribution
Within cells and tissues, this compound is transported and distributed through interactions with transporters and binding proteins . The compound’s ability to selectively modify thiol groups allows it to accumulate in specific cellular compartments where thiol-containing proteins and peptides are present . This selective distribution can affect the localization and activity of this compound, influencing its overall effectiveness in biochemical analyses .
Subcellular Localization
The subcellular localization of this compound is determined by its interactions with thiol-containing proteins and peptides . The compound can be directed to specific cellular compartments through targeting signals and post-translational modifications . This localization can affect the activity and function of this compound, as it allows the compound to selectively modify thiol groups in specific cellular regions . This targeted modification can have significant effects on cellular function and metabolism, particularly in terms of redox signaling and other processes involving thiol groups .
Preparation Methods
Synthetic Routes and Reaction Conditions: N-(Phenylseleno)phthalimide can be synthesized through the reaction of phthalimide with phenylselenyl chloride in the presence of a base such as triethylamine. The reaction typically occurs in an organic solvent like dichloromethane at room temperature. The product is then purified through recrystallization .
Industrial Production Methods: While specific industrial production methods are not extensively documented, the synthesis generally follows the same principles as laboratory preparation, with adjustments for scale and efficiency. Industrial processes may involve continuous flow reactors and automated purification systems to enhance yield and purity.
Types of Reactions:
Oxidation: this compound can undergo oxidation reactions, forming selenoxide intermediates.
Reduction: It can be reduced to form selenol derivatives.
Substitution: The compound is known for its ability to participate in substitution reactions, particularly with thiols.
Common Reagents and Conditions:
Oxidation: Hydrogen peroxide or m-chloroperbenzoic acid are common oxidizing agents.
Reduction: Sodium borohydride or lithium aluminum hydride are typical reducing agents.
Substitution: Thiol-containing compounds, often in the presence of a base like triethylamine.
Major Products:
Oxidation: Selenoxide derivatives.
Reduction: Selenol derivatives.
Substitution: Thiol-substituted products.
Scientific Research Applications
N-(Phenylseleno)phthalimide has a wide range of applications in scientific research:
Comparison with Similar Compounds
Ebselen (2-phenyl-1,2-benzisoselenazol-3(2H)-one): Another selenamide reagent used for thiol derivatization.
Phenylselenyl chloride: Used in the synthesis of N-(Phenylseleno)phthalimide and other selenenyl compounds.
Diphenyl diselenide: A diselenide compound with similar reactivity towards thiols.
Uniqueness: this compound is unique due to its high selectivity for thiol groups and its ability to promote α-selenenylation reactions. This selectivity and reactivity make it a valuable tool in both synthetic and analytical applications .
Properties
IUPAC Name |
2-phenylselanylisoindole-1,3-dione | |
---|---|---|
Source | PubChem | |
URL | https://pubchem.ncbi.nlm.nih.gov | |
Description | Data deposited in or computed by PubChem | |
InChI |
InChI=1S/C14H9NO2Se/c16-13-11-8-4-5-9-12(11)14(17)15(13)18-10-6-2-1-3-7-10/h1-9H | |
Source | PubChem | |
URL | https://pubchem.ncbi.nlm.nih.gov | |
Description | Data deposited in or computed by PubChem | |
InChI Key |
TYZFQSLJTWPSDS-UHFFFAOYSA-N | |
Source | PubChem | |
URL | https://pubchem.ncbi.nlm.nih.gov | |
Description | Data deposited in or computed by PubChem | |
Canonical SMILES |
C1=CC=C(C=C1)[Se]N2C(=O)C3=CC=CC=C3C2=O | |
Source | PubChem | |
URL | https://pubchem.ncbi.nlm.nih.gov | |
Description | Data deposited in or computed by PubChem | |
Molecular Formula |
C14H9NO2Se | |
Source | PubChem | |
URL | https://pubchem.ncbi.nlm.nih.gov | |
Description | Data deposited in or computed by PubChem | |
DSSTOX Substance ID |
DTXSID20343542 | |
Record name | N-(Phenylseleno)phthalimide | |
Source | EPA DSSTox | |
URL | https://comptox.epa.gov/dashboard/DTXSID20343542 | |
Description | DSSTox provides a high quality public chemistry resource for supporting improved predictive toxicology. | |
Molecular Weight |
302.20 g/mol | |
Source | PubChem | |
URL | https://pubchem.ncbi.nlm.nih.gov | |
Description | Data deposited in or computed by PubChem | |
CAS No. |
71098-88-9 | |
Record name | N-(Phenylseleno)phthalimide | |
Source | EPA DSSTox | |
URL | https://comptox.epa.gov/dashboard/DTXSID20343542 | |
Description | DSSTox provides a high quality public chemistry resource for supporting improved predictive toxicology. | |
Record name | 2-(phenylselanyl)-2,3-dihydro-1H-isoindole-1,3-dione | |
Source | European Chemicals Agency (ECHA) | |
URL | https://echa.europa.eu/information-on-chemicals | |
Description | The European Chemicals Agency (ECHA) is an agency of the European Union which is the driving force among regulatory authorities in implementing the EU's groundbreaking chemicals legislation for the benefit of human health and the environment as well as for innovation and competitiveness. | |
Explanation | Use of the information, documents and data from the ECHA website is subject to the terms and conditions of this Legal Notice, and subject to other binding limitations provided for under applicable law, the information, documents and data made available on the ECHA website may be reproduced, distributed and/or used, totally or in part, for non-commercial purposes provided that ECHA is acknowledged as the source: "Source: European Chemicals Agency, http://echa.europa.eu/". Such acknowledgement must be included in each copy of the material. ECHA permits and encourages organisations and individuals to create links to the ECHA website under the following cumulative conditions: Links can only be made to webpages that provide a link to the Legal Notice page. | |
Retrosynthesis Analysis
AI-Powered Synthesis Planning: Our tool employs the Template_relevance Pistachio, Template_relevance Bkms_metabolic, Template_relevance Pistachio_ringbreaker, Template_relevance Reaxys, Template_relevance Reaxys_biocatalysis model, leveraging a vast database of chemical reactions to predict feasible synthetic routes.
One-Step Synthesis Focus: Specifically designed for one-step synthesis, it provides concise and direct routes for your target compounds, streamlining the synthesis process.
Accurate Predictions: Utilizing the extensive PISTACHIO, BKMS_METABOLIC, PISTACHIO_RINGBREAKER, REAXYS, REAXYS_BIOCATALYSIS database, our tool offers high-accuracy predictions, reflecting the latest in chemical research and data.
Strategy Settings
Precursor scoring | Relevance Heuristic |
---|---|
Min. plausibility | 0.01 |
Model | Template_relevance |
Template Set | Pistachio/Bkms_metabolic/Pistachio_ringbreaker/Reaxys/Reaxys_biocatalysis |
Top-N result to add to graph | 6 |
Feasible Synthetic Routes
Q1: What is the primary mode of action of N-(Phenylseleno)phthalimide (NPSP)?
A1: this compound functions as a versatile electrophilic phenylselenium (SePh) donor. [] It selectively reacts with thiols, making it useful for thiol protein/peptide analysis. [, ] The reactivity of NPSP towards thiols can reveal information about the thiol's chemical environment within a peptide and its solvent accessibility within a protein. []
Q2: Can you describe the structural characteristics of NPSP?
A2: Although the provided abstracts do not specify the exact molecular formula, weight, or spectroscopic data for NPSP, its structure can be inferred from its name and reactions. It is a phthalimide derivative containing a phenylseleno group attached to the nitrogen atom.
Q3: Which reactions is NPSP commonly used in?
A3: NPSP finds extensive use in various organic reactions, including:
- α-Selenenylation of aldehydes and ketones: NPSP acts as a selenylating agent in these reactions, often catalyzed by L-prolinamide or pyrrolidine trifluoromethanesulfonamide. This leads to the formation of α-phenylselenoaldehydes and α-phenylseleno ketones. [, , , ]
- C-Se cross-coupling reactions: Silver(I)-catalyzed reactions using NPSP facilitate the phenylselenylation of terminal alkynes and organoboronic acids, yielding alkynyl and diaryl selenides. []
- Amidoselenenylation of alkenes: In the presence of a catalyst like TiCl4, NPSP serves as both a nitrogen and selenium source, enabling the formation of vicinal amidoselenides from alkenes. [, ]
- Hydroxyselenylation and silanoxyselenylation of allylic silanols: NPSP mediates both reactions, with regioselectivity dictated by reaction conditions (basic vs. acidic). []
- Cysteine-selective peptide modification: NPSP reacts with free cysteine thiols, attaching a benzeneselenol (SePh) group. This modification aids in cysteine-selective proteomics. []
Q4: What catalysts are often employed in conjunction with NPSP?
A4: Several catalysts are reported to enhance NPSP's reactivity:
- Organocatalysts: L-prolinamide [, ] and pyrrolidine trifluoromethanesulfonamide [] are effective for α-selenenylation of aldehydes and ketones, respectively.
- Lewis Acids: Silver(I) catalysts promote C-Se cross-coupling reactions. []
- Transition Metal Catalysts: TiCl4 catalyzes intermolecular amidoselenenylation of alkenes. [, ]
Q5: How does the structure of NPSP contribute to its reactivity in α-selenenylation reactions?
A5: While specific structure-activity relationship studies on NPSP are not detailed in the abstracts, computational studies using ab initio methods and density functional theory on L-proline and L-prolinamide catalyzed α-selenenylation reactions with NPSP reveal mechanistic insights:
- The rate-limiting step involves the enamine intermediate attacking the selenium atom in NPSP. []
- The prolinamide-catalyzed reaction has a lower transition state energy compared to the proline-catalyzed reaction, explaining its higher experimental efficiency. []
- Hydrogen bonding plays a crucial role in stabilizing transition states during the reaction. []
Q6: Are there specific analytical techniques used to study NPSP and its reactions?
A6:
- Mass Spectrometry (MS): NPSP derivatization, coupled with on-column digestion and electrochemical reduction, enables rapid bottom-up proteomics. [] Tandem MS analysis is utilized to study selenamide-derivatized peptide ions. [] UVPD (ultraviolet photodissociation) at 266 nm selectively cleaves the S-Se bond in NPSP-tagged peptides, facilitating cysteine-selective peptide identification. [, ]
- Gas Chromatography-Mass Spectrometry (GC/MS): Used to analyze NPSP-treated samples, such as basil plants cultivated using different farming practices. []
Q7: Have any alternatives or substitutes for NPSP been explored in the reported research?
A7: While the abstracts do not directly compare NPSP with alternative reagents, the use of N-bromosuccinimide (NBS) in conjunction with quaternary ammonium halides for generating mixed halogens for halolactonization is mentioned. [] This suggests potential alternative pathways for achieving similar transformations.
Q8: What are the limitations of using NPSP?
A8: One identified limitation involves its use with tri-n-butylphosphine for converting alcohols to selenides, where 3-phthalimido derivative formation is observed in allylic 3-sterols. [] This highlights potential side reactions and the need for careful consideration of substrate structure.
Disclaimer and Information on In-Vitro Research Products
Please be aware that all articles and product information presented on BenchChem are intended solely for informational purposes. The products available for purchase on BenchChem are specifically designed for in-vitro studies, which are conducted outside of living organisms. In-vitro studies, derived from the Latin term "in glass," involve experiments performed in controlled laboratory settings using cells or tissues. It is important to note that these products are not categorized as medicines or drugs, and they have not received approval from the FDA for the prevention, treatment, or cure of any medical condition, ailment, or disease. We must emphasize that any form of bodily introduction of these products into humans or animals is strictly prohibited by law. It is essential to adhere to these guidelines to ensure compliance with legal and ethical standards in research and experimentation.