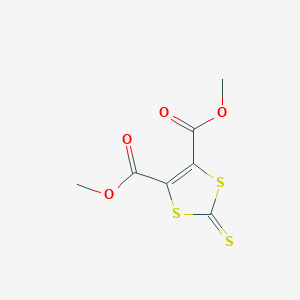
Dimethyl 2-thioxo-1,3-dithiole-4,5-dicarboxylate
Overview
Description
Synthesis Analysis
The synthesis of dimethyl 2-thioxo-1,3-dithiole-4,5-dicarboxylate involves the conversion of 2-thioxo-1,3-dithiol-4,5-dicarboxylic acid methyl ester into various derivatives through processes such as oxidative rearrangement-cyclization. This process leads to the formation of new heterocyclic systems, including (4H,6H)-1,3-dithiolo[4,5-d]pyrimidine-2,5,7-trione, demonstrating the compound's role as a versatile synthetic intermediate (Neiland et al., 1992).
Molecular Structure Analysis
The molecular structure of this compound and its derivatives has been explored through X-ray crystallography, revealing differences in conformational behavior influenced by substituents at the nitrogen atom. For example, N,N-dimethyl 5-(methylthio)-2-thioxo-1,3-dithiole-4-carboxamide demonstrates a lack of resonance effect along the α-oxoketene dithioacetal fragment in the absence of an aromatic system (Heinemann et al., 1995).
Chemical Reactions and Properties
This compound participates in cycloaddition reactions, illustrating its reactivity and potential for creating new heterocyclic structures. The reaction with dimethyl acetylenedicarboxylate under irradiation conditions signifies its capability to undergo efficient cycloadditions, leading to novel fluorinated thiaheterocycles through unique reaction mechanisms (Timoshenko et al., 2003).
Physical Properties Analysis
The physical properties of this compound derivatives, such as crystal structure and spectroscopic characterizations (FT-IR, 1H, and 13C NMR), have been comprehensively studied. These analyses provide insight into the compound's structural features and the influence of different substituents on its physical characteristics. For instance, the structural elucidation of ethyl 2-(dichloromethyl)-4-methyl-1-phenyl-6-thioxo-1,6-dihydropyrimidine-5-carboxylate through X-ray diffraction and spectroscopy demonstrates the detailed examination of its physical attributes (Pekparlak et al., 2018).
Scientific Research Applications
Dimethyl 2-thioxo-1,3-dithiole-4,5-dicarboxylate has been used in the synthesis of new heterocyclic systems, such as (4H,6H)-1,3-dithiolo[4,5-d]pyrimidine-2,5,7-trione, and previously unknown 5,6-dimercaptouracil derivatives, including nickel dithiolene complex (Neiland et al., 1992).
Reactions of 1,2-dithiole-3-thiones and 1,3-dithiolan-2-thiones with acetylenic esters and benzyne have been explored, leading to the formation of adducts containing 2-thioacylmethylene-1,3-dithiole systems (Easton et al., 1972).
The crystal structures of two closely related compounds, N,N-dimethyl 5-(methylthio)-2-thioxo-1,3-dithiole-4-carboxamide and N-(p-methoxy-phenyl)-N-methyl 5-(methylthio)-2-thioxo-1,3-dithiole-4-carboxamide, have been characterized, showing significant differences in conformational behavior related to the nature of the substituents (Heinemann et al., 1995).
The cycloaddition reaction between dimethyl 2-(1-fluoro-2-trifluoromethyl-2-thioxoethylidene)-1,3-dithiole-4,5-dicarboxylate and dimethyl acetylenedicarboxylate was studied, leading to the formation of new fluorinated thiaheterocycles (Timoshenko et al., 2003).
The synthesis of dialkyl 2-[4,5-bis(alkoxycarbonyl)-2-(aryl{alkyl}imino)-3(2H)-thienylidene]-1,3-dithiole-4,5-dicarboxylates through a new cycloaddition reaction of 2-chloroprop-2-enethioamides with activated alkynes was demonstrated, showing a novel reaction pathway (Ogurtsov & Rakitin, 2022).
Mechanism of Action
Mode of Action
The exact mode of action of Dimethyl 2-thioxo-1,3-dithiole-4,5-dicarboxylate is currently unknown due to the lack of comprehensive studies on this compound. It is a sulphur-containing heterocyclic building block , which suggests that it may interact with its targets through the formation of covalent bonds, given the reactivity of sulfur atoms.
Biochemical Pathways
It has been reported to be formed during the reaction of 1,3-dithiolan-2-thiones with dimethyl acetylenedicarboxylate . This suggests that it might be involved in the biochemical pathways related to these reactions.
properties
IUPAC Name |
dimethyl 2-sulfanylidene-1,3-dithiole-4,5-dicarboxylate | |
---|---|---|
Source | PubChem | |
URL | https://pubchem.ncbi.nlm.nih.gov | |
Description | Data deposited in or computed by PubChem | |
InChI |
InChI=1S/C7H6O4S3/c1-10-5(8)3-4(6(9)11-2)14-7(12)13-3/h1-2H3 | |
Source | PubChem | |
URL | https://pubchem.ncbi.nlm.nih.gov | |
Description | Data deposited in or computed by PubChem | |
InChI Key |
UZKKFHMMXWDIPD-UHFFFAOYSA-N | |
Source | PubChem | |
URL | https://pubchem.ncbi.nlm.nih.gov | |
Description | Data deposited in or computed by PubChem | |
Canonical SMILES |
COC(=O)C1=C(SC(=S)S1)C(=O)OC | |
Source | PubChem | |
URL | https://pubchem.ncbi.nlm.nih.gov | |
Description | Data deposited in or computed by PubChem | |
Molecular Formula |
C7H6O4S3 | |
Source | PubChem | |
URL | https://pubchem.ncbi.nlm.nih.gov | |
Description | Data deposited in or computed by PubChem | |
DSSTOX Substance ID |
DTXSID00338259 | |
Record name | Dimethyl 2-thioxo-1,3-dithiole-4,5-dicarboxylate | |
Source | EPA DSSTox | |
URL | https://comptox.epa.gov/dashboard/DTXSID00338259 | |
Description | DSSTox provides a high quality public chemistry resource for supporting improved predictive toxicology. | |
Molecular Weight |
250.3 g/mol | |
Source | PubChem | |
URL | https://pubchem.ncbi.nlm.nih.gov | |
Description | Data deposited in or computed by PubChem | |
CAS RN |
7396-41-0 | |
Record name | Dimethyl 2-thioxo-1,3-dithiole-4,5-dicarboxylate | |
Source | EPA DSSTox | |
URL | https://comptox.epa.gov/dashboard/DTXSID00338259 | |
Description | DSSTox provides a high quality public chemistry resource for supporting improved predictive toxicology. | |
Retrosynthesis Analysis
AI-Powered Synthesis Planning: Our tool employs the Template_relevance Pistachio, Template_relevance Bkms_metabolic, Template_relevance Pistachio_ringbreaker, Template_relevance Reaxys, Template_relevance Reaxys_biocatalysis model, leveraging a vast database of chemical reactions to predict feasible synthetic routes.
One-Step Synthesis Focus: Specifically designed for one-step synthesis, it provides concise and direct routes for your target compounds, streamlining the synthesis process.
Accurate Predictions: Utilizing the extensive PISTACHIO, BKMS_METABOLIC, PISTACHIO_RINGBREAKER, REAXYS, REAXYS_BIOCATALYSIS database, our tool offers high-accuracy predictions, reflecting the latest in chemical research and data.
Strategy Settings
Precursor scoring | Relevance Heuristic |
---|---|
Min. plausibility | 0.01 |
Model | Template_relevance |
Template Set | Pistachio/Bkms_metabolic/Pistachio_ringbreaker/Reaxys/Reaxys_biocatalysis |
Top-N result to add to graph | 6 |
Feasible Synthetic Routes
Q & A
Q1: How is Dimethyl 2-thioxo-1,3-dithiole-4,5-dicarboxylate synthesized?
A1: this compound is formed through the reaction of a 1,3-dithiolan-2-thione with dimethyl acetylenedicarboxylate. [] This reaction also results in the elimination of an olefin molecule. []
Q2: What is the significance of this synthetic route in the context of the research?
A2: This specific reaction pathway highlights the distinct reactivity differences between 1,2-dithiole-3-thiones and 1,3-dithiolan-2-thiones with acetylenic esters. [] While 1,2-dithiole-3-thiones primarily form adducts incorporating the acetylenic structure, 1,3-dithiolan-2-thiones undergo a more profound transformation, leading to the formation of this compound. [] This difference in reactivity provides valuable insights into the chemical behavior of these sulfur-containing heterocycles.
Disclaimer and Information on In-Vitro Research Products
Please be aware that all articles and product information presented on BenchChem are intended solely for informational purposes. The products available for purchase on BenchChem are specifically designed for in-vitro studies, which are conducted outside of living organisms. In-vitro studies, derived from the Latin term "in glass," involve experiments performed in controlled laboratory settings using cells or tissues. It is important to note that these products are not categorized as medicines or drugs, and they have not received approval from the FDA for the prevention, treatment, or cure of any medical condition, ailment, or disease. We must emphasize that any form of bodily introduction of these products into humans or animals is strictly prohibited by law. It is essential to adhere to these guidelines to ensure compliance with legal and ethical standards in research and experimentation.