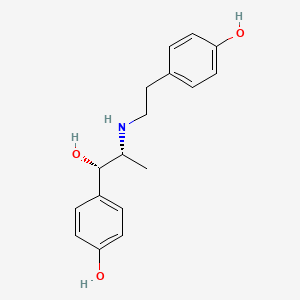
Ritodrine
Overview
Description
Ritodrine is a phenethylamine derivative with tocolytic activity . It binds to and activates beta-2 adrenergic receptors of myometrial cells in the uterus, which decreases the intensity and frequency of uterine contractions .
Synthesis Analysis
Ritodrine is a short-acting β 2-agonist with a hydroxyphenol group on a basic nitrogen forming a hydrogen bond . Since ritodrine has a bulky N-substituent, it has high β 2 selectivity .
Molecular Structure Analysis
The molecular formula of Ritodrine is C17H21NO3 . It is a phenethylamine derivative .
Chemical Reactions Analysis
A rapid and sensitive spectrophotometric method is described for the determination of ritodrine hydrochloride (RTH) and amoxicillin (AMX) in both pure and dosage forms . The proposed method uses 3-methyl benzothiazolin-2-one hydrazone as a chromogenic reagent .
Physical And Chemical Properties Analysis
Ritodrine has a molecular weight of 287.35 g/mol . It is a phenethylamine derivative with tocolytic activity .
Scientific Research Applications
Preterm Labor Management
Ritodrine is primarily used as a tocolytic agent to manage preterm labor. It works by stimulating beta-2 adrenergic receptors, leading to a decrease in intracellular calcium concentration and relaxation of uterine smooth muscle . This action helps in reducing premature uterine contractions, thereby delaying preterm birth.
Genetic Research in Preterm Labor
Studies have investigated the effects of β2-adrenergic receptor gene polymorphisms on Ritodrine therapy outcomes in pregnant women with preterm labor . Research in this area can help understand individual responses to Ritodrine and potentially guide personalized medicine approaches for managing preterm labor.
Adverse Event Prediction
Research has also focused on the associations of GNAS and RGS gene polymorphisms with the risk of Ritodrine-induced adverse events . This is crucial for developing risk scoring systems to identify high-risk patients and improve the safety profile of Ritodrine therapy.
External Cephalic Version (ECV)
Ritodrine has been studied for its effectiveness and safety in external cephalic version (ECV) procedures . ECV is a process by which a breech baby is turned to the head-down position before labor begins, and Ritodrine’s muscle relaxation properties may improve the success rates of this procedure.
Mechanism of Action
Ritodrine is a medication primarily used to manage premature labor. It operates as a beta-2 adrenergic agonist, which means it interacts with beta-2 adrenergic receptors in the body . Here is a detailed overview of its mechanism of action:
Target of Action
Ritodrine primarily targets the Beta-2 adrenergic receptors . These receptors are located at sympathetic neuroeffector junctions of many organs, including the uterus . By interacting with these receptors, Ritodrine can influence the contraction of the uterine smooth muscle .
Mode of Action
Ritodrine binds to the beta-2 adrenergic receptors on the outer membrane of myometrial cells . This binding activates an enzyme called adenyl cyclase, which increases the level of cyclic adenosine monophosphate (cAMP) within the cell . The increase in cAMP subsequently decreases the concentration of intracellular calcium . This decrease in calcium concentration leads to a relaxation of the uterine smooth muscle, thereby reducing premature uterine contractions .
Biochemical Pathways
The primary biochemical pathway affected by Ritodrine involves the cAMP pathway . The activation of adenyl cyclase and the subsequent increase in cAMP levels are key steps in this pathway . The elevated cAMP levels lead to a decrease in intracellular calcium concentrations, which ultimately results in the relaxation of uterine smooth muscle .
Result of Action
The primary molecular effect of Ritodrine’s action is the decrease in intracellular calcium concentration . This decrease leads to the relaxation of uterine smooth muscle, which is the primary cellular effect . As a result, Ritodrine effectively decreases premature uterine contractions .
Action Environment
Environmental factors can influence the action, efficacy, and stability of Ritodrine. For instance, genetic polymorphisms of the ADRB2 gene, which encodes the Beta-2 adrenergic receptor, could affect the therapy outcomes of Ritodrine in patients with preterm labor . .
Safety and Hazards
Ritodrine may be useful for the short-term prolongation of pregnancy, but the high incidence of adverse reactions means that administration should be limited . It is recommended to avoid dust formation, avoid breathing mist, gas or vapours, avoid contacting with skin and eye, use personal protective equipment, wear chemical impermeable gloves, ensure adequate ventilation, remove all sources of ignition, evacuate personnel to safe areas, and keep people away from and upwind of spill/leak .
Future Directions
properties
IUPAC Name |
4-[2-[[(1S,2R)-1-hydroxy-1-(4-hydroxyphenyl)propan-2-yl]amino]ethyl]phenol | |
---|---|---|
Source | PubChem | |
URL | https://pubchem.ncbi.nlm.nih.gov | |
Description | Data deposited in or computed by PubChem | |
InChI |
InChI=1S/C17H21NO3/c1-12(17(21)14-4-8-16(20)9-5-14)18-11-10-13-2-6-15(19)7-3-13/h2-9,12,17-21H,10-11H2,1H3/t12-,17-/m1/s1 | |
Source | PubChem | |
URL | https://pubchem.ncbi.nlm.nih.gov | |
Description | Data deposited in or computed by PubChem | |
InChI Key |
IOVGROKTTNBUGK-SJKOYZFVSA-N | |
Source | PubChem | |
URL | https://pubchem.ncbi.nlm.nih.gov | |
Description | Data deposited in or computed by PubChem | |
Canonical SMILES |
CC(C(C1=CC=C(C=C1)O)O)NCCC2=CC=C(C=C2)O | |
Source | PubChem | |
URL | https://pubchem.ncbi.nlm.nih.gov | |
Description | Data deposited in or computed by PubChem | |
Isomeric SMILES |
C[C@H]([C@H](C1=CC=C(C=C1)O)O)NCCC2=CC=C(C=C2)O | |
Source | PubChem | |
URL | https://pubchem.ncbi.nlm.nih.gov | |
Description | Data deposited in or computed by PubChem | |
Molecular Formula |
C17H21NO3 | |
Source | PubChem | |
URL | https://pubchem.ncbi.nlm.nih.gov | |
Description | Data deposited in or computed by PubChem | |
Molecular Weight |
287.35 g/mol | |
Source | PubChem | |
URL | https://pubchem.ncbi.nlm.nih.gov | |
Description | Data deposited in or computed by PubChem | |
Product Name |
Yutopar | |
CAS RN |
26652-09-5 | |
Record name | Ritodrine | |
Source | CAS Common Chemistry | |
URL | https://commonchemistry.cas.org/detail?cas_rn=26652-09-5 | |
Description | CAS Common Chemistry is an open community resource for accessing chemical information. Nearly 500,000 chemical substances from CAS REGISTRY cover areas of community interest, including common and frequently regulated chemicals, and those relevant to high school and undergraduate chemistry classes. This chemical information, curated by our expert scientists, is provided in alignment with our mission as a division of the American Chemical Society. | |
Explanation | The data from CAS Common Chemistry is provided under a CC-BY-NC 4.0 license, unless otherwise stated. | |
Record name | Ritodrine [USAN:INN:BAN] | |
Source | ChemIDplus | |
URL | https://pubchem.ncbi.nlm.nih.gov/substance/?source=chemidplus&sourceid=0026652095 | |
Description | ChemIDplus is a free, web search system that provides access to the structure and nomenclature authority files used for the identification of chemical substances cited in National Library of Medicine (NLM) databases, including the TOXNET system. | |
Record name | Ritodrine | |
Source | European Chemicals Agency (ECHA) | |
URL | https://echa.europa.eu/substance-information/-/substanceinfo/100.043.512 | |
Description | The European Chemicals Agency (ECHA) is an agency of the European Union which is the driving force among regulatory authorities in implementing the EU's groundbreaking chemicals legislation for the benefit of human health and the environment as well as for innovation and competitiveness. | |
Explanation | Use of the information, documents and data from the ECHA website is subject to the terms and conditions of this Legal Notice, and subject to other binding limitations provided for under applicable law, the information, documents and data made available on the ECHA website may be reproduced, distributed and/or used, totally or in part, for non-commercial purposes provided that ECHA is acknowledged as the source: "Source: European Chemicals Agency, http://echa.europa.eu/". Such acknowledgement must be included in each copy of the material. ECHA permits and encourages organisations and individuals to create links to the ECHA website under the following cumulative conditions: Links can only be made to webpages that provide a link to the Legal Notice page. | |
Record name | RITODRINE | |
Source | FDA Global Substance Registration System (GSRS) | |
URL | https://gsrs.ncats.nih.gov/ginas/app/beta/substances/I0Q6O6740J | |
Description | The FDA Global Substance Registration System (GSRS) enables the efficient and accurate exchange of information on what substances are in regulated products. Instead of relying on names, which vary across regulatory domains, countries, and regions, the GSRS knowledge base makes it possible for substances to be defined by standardized, scientific descriptions. | |
Explanation | Unless otherwise noted, the contents of the FDA website (www.fda.gov), both text and graphics, are not copyrighted. They are in the public domain and may be republished, reprinted and otherwise used freely by anyone without the need to obtain permission from FDA. Credit to the U.S. Food and Drug Administration as the source is appreciated but not required. | |
Retrosynthesis Analysis
AI-Powered Synthesis Planning: Our tool employs the Template_relevance Pistachio, Template_relevance Bkms_metabolic, Template_relevance Pistachio_ringbreaker, Template_relevance Reaxys, Template_relevance Reaxys_biocatalysis model, leveraging a vast database of chemical reactions to predict feasible synthetic routes.
One-Step Synthesis Focus: Specifically designed for one-step synthesis, it provides concise and direct routes for your target compounds, streamlining the synthesis process.
Accurate Predictions: Utilizing the extensive PISTACHIO, BKMS_METABOLIC, PISTACHIO_RINGBREAKER, REAXYS, REAXYS_BIOCATALYSIS database, our tool offers high-accuracy predictions, reflecting the latest in chemical research and data.
Strategy Settings
Precursor scoring | Relevance Heuristic |
---|---|
Min. plausibility | 0.01 |
Model | Template_relevance |
Template Set | Pistachio/Bkms_metabolic/Pistachio_ringbreaker/Reaxys/Reaxys_biocatalysis |
Top-N result to add to graph | 6 |
Feasible Synthetic Routes
Disclaimer and Information on In-Vitro Research Products
Please be aware that all articles and product information presented on BenchChem are intended solely for informational purposes. The products available for purchase on BenchChem are specifically designed for in-vitro studies, which are conducted outside of living organisms. In-vitro studies, derived from the Latin term "in glass," involve experiments performed in controlled laboratory settings using cells or tissues. It is important to note that these products are not categorized as medicines or drugs, and they have not received approval from the FDA for the prevention, treatment, or cure of any medical condition, ailment, or disease. We must emphasize that any form of bodily introduction of these products into humans or animals is strictly prohibited by law. It is essential to adhere to these guidelines to ensure compliance with legal and ethical standards in research and experimentation.