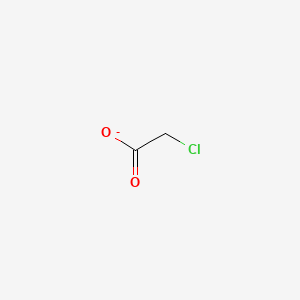
Chloroacetate
Overview
Description
Chloroacetate (ClCH₂COO⁻) is a halogenated carboxylate with widespread applications in chemical synthesis, pharmaceuticals, and agrochemicals. Its sodium salt (sodium this compound) is a white, water-soluble solid used in herbicides, dyes, and drug synthesis . This compound also serves as a critical intermediate in the biodegradation of chlorinated pollutants like chloroethenes .
Scientific Research Applications
Agricultural Applications
Herbicides and Pesticides
Chloroacetate derivatives are widely used in the formulation of herbicides and pesticides. For example, sodium this compound serves as a formulation component in herbicides targeting weeds during the seedling stages of crops such as Brussels sprouts and kale . Its effectiveness in controlling weed growth makes it a valuable tool in agricultural practices.
Chemical Intermediate
Chloroacetates are also utilized as chemical intermediates in the production of various agrochemicals. They play a crucial role in synthesizing compounds that protect crops from pests and diseases, thereby enhancing agricultural productivity .
Pharmaceutical Applications
Synthesis of Active Pharmaceutical Ingredients
Chloroacetates are important intermediates in the synthesis of several pharmaceutical compounds. For instance, methyl this compound is used in the production of various drugs due to its reactivity and ability to introduce chloroacetyl groups into organic molecules . This property is essential for developing medications that require specific functional groups for biological activity.
Enzyme Activity Studies
Recent studies have highlighted the use of this compound esterase in histochemical methods to detect enzyme activity within specific tissue microenvironments. This application is particularly relevant in immunohistochemistry, where this compound esterase can help characterize mast cell populations and their functional significance in various diseases .
Chemical Synthesis
Solvent for Organic Reactions
Chloroacetates, such as ethyl this compound, are commonly used as solvents in organic synthesis. They facilitate various reactions due to their ability to dissolve a wide range of organic compounds . Their role as solvents is critical in laboratory settings for synthesizing complex organic molecules.
Intermediate in Organic Synthesis
Chloroacetates serve as intermediates in the production of other chemicals, including pesticides and pharmaceuticals. Their utility in generating diverse chemical structures makes them indispensable in synthetic organic chemistry .
Analytical Chemistry
Detection and Quantification
this compound compounds are employed in analytical chemistry for detecting and quantifying various substances. Their reactivity allows them to form derivatives that can be analyzed using techniques such as gas chromatography and mass spectrometry . This application is crucial for environmental monitoring and quality control in chemical manufacturing.
Summary Table of Applications
Application Area | Specific Use | Example Compounds |
---|---|---|
Agricultural | Herbicides and pesticides | Sodium this compound |
Pharmaceutical | Synthesis of active pharmaceutical ingredients | Methyl this compound |
Chemical Synthesis | Solvent for organic reactions | Ethyl this compound |
Analytical Chemistry | Detection and quantification | Chloroacetic acid derivatives |
Chemical Reactions Analysis
Chemical Reactions Involving Chloroacetate
This compound participates in several important chemical reactions, which can be categorized into esterification, hydrolysis, and substitution reactions.
Esterification Reactions
This compound can react with alcohols to form esters. The reaction typically requires an acid catalyst or a Lewis acid to facilitate the process.
-
Example Reaction :
-
Research Findings : An investigation into the esterification of chloroacetic acid with methanol showed that using a cation exchange resin as a catalyst resulted in a conversion rate of approximately 70.11% under optimal conditions (1.4:1 alcohol/acid molar ratio, 70 °C) .
Hydrolysis Reactions
This compound can undergo hydrolysis to produce glycolic acid, particularly when reacted with water under acidic or basic conditions.
-
Example Reaction :
Substitution Reactions
As a haloacetate, this compound can participate in nucleophilic substitution reactions where it acts as an electrophile.
-
Example Reaction :
The reaction of this compound with nucleophiles (e.g., amines or thiols) can lead to the formation of various derivatives. -
Research Findings : A study demonstrated that chloroacetates react with substituted epoxides in the presence of lithium salts or zinc chloride to yield complex products through normal and abnormal cleavage pathways .
Table 2: Reaction Conditions for Esterification of Chloroacetic Acid
Alcohol | Catalyst Type | Molar Ratio (Alcohol:Acid) | Temperature (°C) | Conversion Rate (%) |
---|---|---|---|---|
Methanol | Cation Exchange Resin | 1.4:1 | 70 | 70.11 |
Isopropanol | Lanthanum Dodecyl Sulfate (LDDS) | 1.2:1 | Reflux | 98.3 |
Q & A
Q. Basic: What are the recommended laboratory safety protocols for handling sodium chloroacetate in synthetic chemistry experiments?
Answer:
Sodium this compound (C₂H₃ClO₂·Na) requires stringent safety measures due to its acute toxicity (oral, dermal) and irritant properties. Key protocols include:
- Personal Protective Equipment (PPE): Wear NIOSH/MSHA-approved respirators, chemical-resistant gloves (e.g., nitrile), and OSHA-compliant goggles to prevent inhalation, skin contact, or ocular exposure .
- First Aid: Immediate flushing of eyes (10–15 mins with water) and skin (soap and water for 15 mins) is critical. Contaminated clothing must be washed before reuse .
- Ventilation: Use fume hoods for powder handling to avoid airborne dispersion.
Q. Basic: How can researchers optimize the synthesis of sodium this compound from chloroacetic acid while minimizing byproducts?
Answer:
Synthesis typically involves neutralizing chloroacetic acid with sodium hydroxide. Methodological considerations:
- Stoichiometric Control: Maintain a 1:1 molar ratio of chloroacetic acid to NaOH to avoid excess base, which may hydrolyze this compound to glycolate.
- Temperature: Conduct reactions at 0–5°C to suppress side reactions (e.g., dithis compound formation).
- Purification: Recrystallize from ethanol/water mixtures (80:20 v/v) to achieve ≥98% purity, verified via HPLC with UV detection (λ = 210 nm) .
Q. Advanced: How can contradictory toxicity data between in vitro and in vivo models for sodium this compound be resolved?
Answer:
Discrepancies often arise from metabolic differences (e.g., hepatic conversion to glycine derivatives in vivo). Strategies:
- Dose-Response Analysis: Compare LD₅₀ values (e.g., 320 mg/kg in rats ) with in vitro IC₅₀ in hepatocyte assays.
- Metabolite Tracking: Use LC-MS to identify species-specific metabolites (e.g., thioether conjugates in rodents).
- Cross-Species Modeling: Apply physiologically based pharmacokinetic (PBPK) models to extrapolate human toxicity thresholds .
Q. Advanced: What experimental designs are suitable for studying the pH-dependent stability of this compound in aqueous solutions?
Answer:
A factorial design approach is recommended to evaluate variables:
Factor | Levels | Response Variable |
---|---|---|
pH | 3.0, 5.0, 7.0, 9.0 | Degradation rate (k, h⁻¹) |
Temperature (°C) | 25, 40, 60 | Half-life (t₁/₂) |
Ionic Strength (M) | 0.1, 0.5, 1.0 | Byproduct concentration (%) |
- Analytical Method: Monitor degradation via ion chromatography (IC) with conductivity detection.
- Findings: this compound is most stable at pH 5–7 (t₁/₂ > 48 hrs at 25°C), with accelerated hydrolysis under alkaline conditions (pH >9) forming glycolate .
Q. Basic: What analytical techniques are validated for quantifying trace impurities in sodium this compound batches?
Answer:
- HPLC-UV: C18 column, mobile phase = 0.1% H₃PO₄ in acetonitrile/water (30:70), detects dithis compound (<0.1% w/w) .
- ICP-MS: Quantifies heavy metals (e.g., Pb, Hg) at ppb levels, critical for pharmaceutical-grade synthesis .
- Karl Fischer Titration: Determines moisture content (<0.5% w/w) to prevent hydrolysis during storage .
Q. Advanced: How can mechanistic studies elucidate the role of this compound in disrupting cellular glutathione (GSH) pathways?
Answer:
- In Vitro Models: Expose HepG2 cells to 0.1–10 mM this compound and measure GSH depletion via Ellman’s assay.
- Enzyme Inhibition Assays: Test this compound’s affinity for glutathione-S-transferase (GST) using recombinant enzymes (Km and Vmax calculations) .
- Omics Integration: Pair metabolomics (GC-MS) with transcriptomics to map Nrf2-mediated antioxidant response pathways .
Q. Basic: What are the regulatory thresholds for this compound in environmental water samples, and how are they validated?
Answer:
- EPA Guidelines: Maximum contaminant level (MCL) = 0.07 mg/L, based on chronic toxicity in aquatic organisms .
- Validation: Use spike-and-recovery experiments with surface water samples. Acceptable recovery = 85–115%, RSD <10% via EPA Method 552.3 .
Q. Advanced: What computational methods predict the reactivity of this compound in nucleophilic substitution reactions?
Answer:
- DFT Calculations: Optimize transition states (e.g., SN2 displacement at the α-carbon) using B3LYP/6-311++G(d,p) basis sets.
- Solvent Effects: Apply COSMO-RS to simulate reaction kinetics in polar aprotic solvents (e.g., DMF vs. DMSO) .
- Validation: Compare computed activation energies (ΔG‡) with experimental Arrhenius parameters from kinetic studies .
Comparison with Similar Compounds
Structural and Functional Analogs of Chloroacetate
This compound belongs to a family of haloacetates, including fluoro-, bromo-, iodo-, and dithis compound. Key comparisons are outlined below:
Enzymatic Degradation and Kinetic Properties
- Fluoroacetate (FCH₂COO⁻) : Fluoroacetate dehalogenase degrades fluoroacetate with a 10-fold higher substrate affinity (Kₐₜ = 2.4 × 10⁻⁵ M) compared to this compound (Kₐₜ = 2.0 × 10⁻⁴ M). However, this compound exhibits a 6-fold lower Vmax .
- Bromoacetate (BrCH₂COO⁻) and Iodoacetate (ICH₂COO⁻) : Both are degraded by the same enzyme but with lower efficiency than fluoroacetate .
Table 1: Kinetic Parameters of Haloacetate Degradation by Fluoroacetate Dehalogenase
Compound | Kₐₜ (M) | Vmax (Relative) |
---|---|---|
Fluoroacetate | 2.4 × 10⁻⁵ | 6.0 |
This compound | 2.0 × 10⁻⁴ | 1.0 |
Dithis compound (Cl₂CHCOO⁻)
Dithis compound (DCA) shares environmental and therapeutic roles with this compound. While this compound is a biodegradation intermediate, DCA is studied for its metabolic modulation in cancer therapy .
Insecticidal Activity
- Thymyl this compound: A derivative with moderate toxicity (LD₅₀ = 1.41 µg/mg) against leaf-cutting ants (Acromyrmex balzani), compared to thymol (LD₅₀ = 2.23 µg/mg) and deltamethrin (LD₅₀ = 0.87 × 10⁻⁵ µg/mg).
Table 2: Toxicity Profiles of Ant-Control Agents
Compound | LD₅₀ (µg/mg) | Sublethal Effects |
---|---|---|
Deltamethrin | 0.87 × 10⁻⁵ | Rapid mortality |
Thymyl this compound | 1.41 | Altered grooming, disorientation |
Thymol | 2.23 | Reduced foraging, avoidance |
Environmental Impact
This compound is less persistent than dithis compound due to specialized bacterial dehalogenases. Burkholderia species JS666, for instance, harbors duplicated genes for this compound dehalogenase, enhancing its breakdown efficiency .
Properties
CAS No. |
14526-03-5 |
---|---|
Molecular Formula |
C2H2ClO2- |
Molecular Weight |
93.49 g/mol |
IUPAC Name |
2-chloroacetate |
InChI |
InChI=1S/C2H3ClO2/c3-1-2(4)5/h1H2,(H,4,5)/p-1 |
InChI Key |
FOCAUTSVDIKZOP-UHFFFAOYSA-M |
SMILES |
C(C(=O)[O-])Cl |
Canonical SMILES |
C(C(=O)[O-])Cl |
Key on ui other cas no. |
14526-03-5 |
Synonyms |
Acetocaustin chloroacetate chloroacetic acid chloroacetic acid, aluminum salt chloroacetic acid, ammonium (2:1) salt chloroacetic acid, ammonium salt chloroacetic acid, calcium (3:1) salt chloroacetic acid, calcium salt chloroacetic acid, potassium (2:1) salt chloroacetic acid, potassium salt chloroacetic acid, silver salt chloroacetic acid, sodium (2:1) salt chloroacetic acid, sodium (5:2) salt chloroacetic acid, sodium salt monochloroacetic acid SODIUM CHLOROACETATE |
Origin of Product |
United States |
Synthesis routes and methods
Procedure details
Retrosynthesis Analysis
AI-Powered Synthesis Planning: Our tool employs the Template_relevance Pistachio, Template_relevance Bkms_metabolic, Template_relevance Pistachio_ringbreaker, Template_relevance Reaxys, Template_relevance Reaxys_biocatalysis model, leveraging a vast database of chemical reactions to predict feasible synthetic routes.
One-Step Synthesis Focus: Specifically designed for one-step synthesis, it provides concise and direct routes for your target compounds, streamlining the synthesis process.
Accurate Predictions: Utilizing the extensive PISTACHIO, BKMS_METABOLIC, PISTACHIO_RINGBREAKER, REAXYS, REAXYS_BIOCATALYSIS database, our tool offers high-accuracy predictions, reflecting the latest in chemical research and data.
Strategy Settings
Precursor scoring | Relevance Heuristic |
---|---|
Min. plausibility | 0.01 |
Model | Template_relevance |
Template Set | Pistachio/Bkms_metabolic/Pistachio_ringbreaker/Reaxys/Reaxys_biocatalysis |
Top-N result to add to graph | 6 |
Feasible Synthetic Routes
Disclaimer and Information on In-Vitro Research Products
Please be aware that all articles and product information presented on BenchChem are intended solely for informational purposes. The products available for purchase on BenchChem are specifically designed for in-vitro studies, which are conducted outside of living organisms. In-vitro studies, derived from the Latin term "in glass," involve experiments performed in controlled laboratory settings using cells or tissues. It is important to note that these products are not categorized as medicines or drugs, and they have not received approval from the FDA for the prevention, treatment, or cure of any medical condition, ailment, or disease. We must emphasize that any form of bodily introduction of these products into humans or animals is strictly prohibited by law. It is essential to adhere to these guidelines to ensure compliance with legal and ethical standards in research and experimentation.