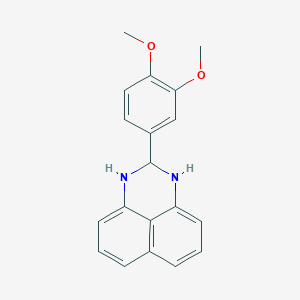
2-(3,4-dimethoxyphenyl)-2,3-dihydro-1H-perimidine
- Click on QUICK INQUIRY to receive a quote from our team of experts.
- With the quality product at a COMPETITIVE price, you can focus more on your research.
Overview
Description
2-(3,4-Dimethoxyphenyl)-2,3-dihydro-1H-perimidine is an organic compound that belongs to the class of heterocyclic compounds It is characterized by the presence of a perimidine ring system, which is a fused bicyclic structure containing nitrogen atoms
Preparation Methods
Synthetic Routes and Reaction Conditions
The synthesis of 2-(3,4-dimethoxyphenyl)-2,3-dihydro-1H-perimidine typically involves the condensation of 3,4-dimethoxybenzaldehyde with a suitable amine under acidic or basic conditions. One common method involves the use of ammonium acetate as a catalyst in an ethanol solvent. The reaction mixture is heated under reflux for several hours, leading to the formation of the desired product.
Industrial Production Methods
For industrial-scale production, the synthesis can be optimized by using continuous flow reactors, which allow for better control over reaction conditions and improved yields. The use of microwave-assisted synthesis has also been explored to reduce reaction times and increase efficiency.
Chemical Reactions Analysis
Types of Reactions
2-(3,4-Dimethoxyphenyl)-2,3-dihydro-1H-perimidine undergoes various chemical reactions, including:
Oxidation: The compound can be oxidized to form corresponding quinones.
Reduction: Reduction reactions can convert it into its dihydro derivatives.
Substitution: Electrophilic and nucleophilic substitution reactions can introduce different functional groups onto the aromatic ring.
Common Reagents and Conditions
Oxidation: Common oxidizing agents include potassium permanganate and chromium trioxide.
Reduction: Reducing agents such as sodium borohydride and lithium aluminum hydride are often used.
Substitution: Reagents like halogens, nitrating agents, and sulfonating agents are employed under various conditions.
Major Products Formed
The major products formed from these reactions include quinones, dihydro derivatives, and substituted aromatic compounds, depending on the specific reaction conditions and reagents used.
Scientific Research Applications
Medicinal Chemistry
The perimidine scaffold has been recognized for its potential therapeutic applications due to its biological activities. Studies have shown that derivatives of perimidines exhibit:
- Antimicrobial Activity : Compounds within this class have demonstrated efficacy against various bacterial and fungal strains. The presence of nitrogen atoms in the structure enhances their interaction with biological targets .
- Anticancer Properties : Research indicates that perimidine derivatives can inhibit tumor growth and induce apoptosis in cancer cells. This is attributed to their ability to interfere with cellular signaling pathways .
- Antioxidant Activity : The antioxidant properties of perimidines contribute to their potential use in preventing oxidative stress-related diseases .
Organic Synthesis
2-(3,4-dimethoxyphenyl)-2,3-dihydro-1H-perimidine can be synthesized using various catalytic methods. Recent studies have highlighted the use of nano-catalysts for efficient synthesis:
- Catalytic Efficiency : Nano-γ-Al2O3/BFn and nano-γ-Al2O3/BFn/Fe3O4 have been employed as catalysts for synthesizing substituted perimidines. These catalysts facilitate reactions under mild conditions, leading to high yields and reduced reaction times .
- Green Chemistry Approaches : The synthesis methods often utilize environmentally friendly solvents and conditions, aligning with green chemistry principles. This not only improves safety but also enhances the sustainability of the synthetic processes .
Materials Science
The unique properties of perimidines make them suitable for various applications in materials science:
- Dye Chemistry : Perimidines are utilized as intermediates in dye production due to their ability to form stable complexes with metal ions, resulting in vibrant colors suitable for textiles and polymers .
- Polymer Chemistry : Their incorporation into polymer matrices can enhance material properties such as thermal stability and mechanical strength. This is particularly useful in developing advanced materials for industrial applications .
Theoretical Studies
Recent theoretical studies using density functional theory have provided insights into the chemical reactivity of this compound:
- Reactivity Analysis : Investigations into frontier molecular orbitals reveal that certain nitrogen atoms in the structure are preferred sites for electrophilic attacks, which can guide future synthetic strategies .
- Stability Assessment : The energy gap between molecular orbitals has been correlated with the stability and reactivity of different derivatives, aiding in the design of more effective compounds .
Comprehensive Data Table
Application Area | Specific Use Cases | Key Properties |
---|---|---|
Medicinal Chemistry | Antimicrobial, anticancer, antioxidant | Biological activity against pathogens |
Organic Synthesis | Catalytic synthesis using nano-catalysts | High yield, mild conditions |
Materials Science | Dye production and polymer enhancement | Color stability, improved material properties |
Theoretical Studies | Reactivity analysis using DFT | Insights into stability and reactivity |
Case Studies
Research has documented specific case studies demonstrating the efficacy of this compound:
- Anticancer Activity : A study highlighted the compound's ability to inhibit specific cancer cell lines through apoptosis mechanisms.
- Antimicrobial Efficacy : Another case demonstrated its effectiveness against resistant bacterial strains, showcasing its potential as a lead compound for drug development.
Mechanism of Action
The mechanism of action of 2-(3,4-dimethoxyphenyl)-2,3-dihydro-1H-perimidine involves its interaction with specific molecular targets and pathways. In biological systems, it may interact with enzymes and receptors, leading to modulation of biochemical pathways. The exact molecular targets and pathways are still under investigation, but preliminary studies suggest its potential to inhibit certain enzymes and disrupt cellular processes.
Comparison with Similar Compounds
Similar Compounds
3,4-Dimethoxyphenethylamine: An analogue with similar structural features but different biological activities.
3,4-Dimethoxyphenylacetic acid: Another related compound with distinct chemical properties and applications.
Uniqueness
2-(3,4-Dimethoxyphenyl)-2,3-dihydro-1H-perimidine is unique due to its fused bicyclic structure, which imparts specific chemical and physical properties
Biological Activity
2-(3,4-Dimethoxyphenyl)-2,3-dihydro-1H-perimidine is a derivative of perimidine, a class of compounds known for their diverse biological activities. This article reviews the biological activity of this specific compound, focusing on its synthesis, pharmacological properties, and potential therapeutic applications.
Synthesis of this compound
The synthesis of this compound typically involves the cyclo-condensation of 1,8-naphthalenediamine with 3,4-dimethoxybenzaldehyde. Various methods have been explored to enhance yield and efficiency:
- Catalytic Methods : Utilization of catalysts such as InCl₃ and Brønsted acids has been reported to significantly improve reaction yields under mild conditions .
- Green Chemistry Approaches : Recent advancements emphasize eco-friendly synthesis routes using solid acid catalysts and solvent-free conditions .
Biological Activity Overview
The biological activities of perimidine derivatives, including this compound, encompass a wide range of pharmacological effects:
Antimicrobial Activity
A study evaluating the antimicrobial efficacy of various perimidines found that this compound exhibited significant antibacterial activity against both Gram-positive and Gram-negative bacteria. The Minimum Inhibitory Concentration (MIC) values indicated its potential as an effective antimicrobial agent.
Antitumor Effects
Research conducted on the cytotoxicity of this compound revealed promising results in inducing apoptosis in cancer cell lines such as A549 (lung cancer). The compound showed dose-dependent effects with an IC50 value around 10 µM, suggesting a significant potential for development as an anticancer drug .
Antioxidant Properties
The antioxidant activity was assessed using DPPH radical scavenging assays. Results demonstrated that the compound effectively reduced DPPH radicals, indicating its potential application in oxidative stress-related conditions .
Structure-Activity Relationship (SAR)
The biological activity of this compound can be attributed to its structural features:
- Dimethoxy Substitution : The presence of methoxy groups enhances lipophilicity and may improve interaction with biological membranes.
- Perimidine Core : The nitrogen atoms in the perimidine ring contribute to its electron-rich nature, facilitating interactions with various biological targets.
Properties
Molecular Formula |
C19H18N2O2 |
---|---|
Molecular Weight |
306.4 g/mol |
IUPAC Name |
2-(3,4-dimethoxyphenyl)-2,3-dihydro-1H-perimidine |
InChI |
InChI=1S/C19H18N2O2/c1-22-16-10-9-13(11-17(16)23-2)19-20-14-7-3-5-12-6-4-8-15(21-19)18(12)14/h3-11,19-21H,1-2H3 |
InChI Key |
CRPNVHCEGIQOLN-UHFFFAOYSA-N |
Canonical SMILES |
COC1=C(C=C(C=C1)C2NC3=CC=CC4=C3C(=CC=C4)N2)OC |
Origin of Product |
United States |
Disclaimer and Information on In-Vitro Research Products
Please be aware that all articles and product information presented on BenchChem are intended solely for informational purposes. The products available for purchase on BenchChem are specifically designed for in-vitro studies, which are conducted outside of living organisms. In-vitro studies, derived from the Latin term "in glass," involve experiments performed in controlled laboratory settings using cells or tissues. It is important to note that these products are not categorized as medicines or drugs, and they have not received approval from the FDA for the prevention, treatment, or cure of any medical condition, ailment, or disease. We must emphasize that any form of bodily introduction of these products into humans or animals is strictly prohibited by law. It is essential to adhere to these guidelines to ensure compliance with legal and ethical standards in research and experimentation.