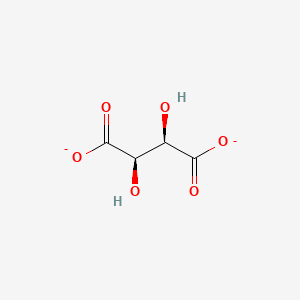
Tartrate ion
Overview
Description
Tartrate ion is a salt or ester of tartaric acid, a naturally occurring organic acid found in various fruits, particularly grapes. The tartrate dianion has the chemical formula C₄H₄O₆²⁻. Tartrate ions are widely used in the food industry as antioxidants, acidity regulators, and emulsifiers. They also play a significant role in pharmaceuticals, where they enhance the stability and absorption of medications .
Preparation Methods
Synthetic Routes and Reaction Conditions
Tartrate ions can be synthesized through the esterification of tartaric acid with alcohols. For example, potassium sodium tartrate can be prepared by reacting tartaric acid with potassium hydroxide and sodium hydroxide under controlled conditions .
Industrial Production Methods
The primary industrial source of tartrates is the wine industry. During wine fermentation, potassium bitartrate precipitates out and is collected from the fermentation vessels. This precipitate is then purified to produce commercial tartrates .
Chemical Reactions Analysis
Types of Reactions
Tartrate ions undergo various chemical reactions, including:
Oxidation: Tartrate ions can be oxidized to produce dihydroxyfumaric acid.
Reduction: Reduction of tartrates can yield tartaric acid.
Complexation: Tartrate ions form strong complexes with transition metals, which are useful in analytical chemistry and catalysis.
Common Reagents and Conditions
Oxidation: Potassium permanganate is commonly used as an oxidizing agent.
Reduction: Sodium borohydride can be used for the reduction of tartrates.
Complexation: Transition metal salts like copper sulfate are used to form metal-tartrate complexes.
Major Products
Oxidation: Dihydroxyfumaric acid.
Reduction: Tartaric acid.
Complexation: Metal-tartrate complexes, such as copper tartrate.
Scientific Research Applications
Tartrate ions have diverse applications in scientific research:
Chemistry: Used in the synthesis of metal-tartrate complexes for catalysis and material science.
Biology: Employed in the study of enzyme inhibition and protein interactions.
Medicine: Used in drug formulations to enhance stability and bioavailability.
Industry: Utilized in the production of adhesives, coatings, and biodegradable polymers.
Mechanism of Action
The mechanism of action of tartrates varies depending on their application. In pharmaceuticals, tartrates act as stabilizers and absorption enhancers. For example, metoprolol tartrate works by blocking beta-1 adrenergic receptors, reducing heart rate and blood pressure . In catalysis, tartrates form complexes with metals, facilitating various chemical reactions .
Comparison with Similar Compounds
Similar Compounds
Citrate: Another salt of a tricarboxylic acid, used similarly in food and pharmaceuticals.
Malate: A salt of malic acid, used in food and beverage industries.
Succinate: A salt of succinic acid, used in biochemistry and pharmaceuticals.
Uniqueness of Tartrate ion
Tartrate ions are unique due to their ability to form strong complexes with transition metals, which is not as pronounced in similar compounds like citrate or malate. This property makes tartrates particularly valuable in catalysis and analytical chemistry .
Properties
IUPAC Name |
(2R,3R)-2,3-dihydroxybutanedioate | |
---|---|---|
Details | Computed by Lexichem TK 2.7.0 (PubChem release 2021.10.14) | |
Source | PubChem | |
URL | https://pubchem.ncbi.nlm.nih.gov | |
Description | Data deposited in or computed by PubChem | |
InChI |
InChI=1S/C4H6O6/c5-1(3(7)8)2(6)4(9)10/h1-2,5-6H,(H,7,8)(H,9,10)/p-2/t1-,2-/m1/s1 | |
Details | Computed by InChI 1.0.6 (PubChem release 2021.10.14) | |
Source | PubChem | |
URL | https://pubchem.ncbi.nlm.nih.gov | |
Description | Data deposited in or computed by PubChem | |
InChI Key |
FEWJPZIEWOKRBE-JCYAYHJZSA-L | |
Details | Computed by InChI 1.0.6 (PubChem release 2021.10.14) | |
Source | PubChem | |
URL | https://pubchem.ncbi.nlm.nih.gov | |
Description | Data deposited in or computed by PubChem | |
Canonical SMILES |
C(C(C(=O)[O-])O)(C(=O)[O-])O | |
Details | Computed by OEChem 2.3.0 (PubChem release 2021.10.14) | |
Source | PubChem | |
URL | https://pubchem.ncbi.nlm.nih.gov | |
Description | Data deposited in or computed by PubChem | |
Isomeric SMILES |
[C@@H]([C@H](C(=O)[O-])O)(C(=O)[O-])O | |
Details | Computed by OEChem 2.3.0 (PubChem release 2021.10.14) | |
Source | PubChem | |
URL | https://pubchem.ncbi.nlm.nih.gov | |
Description | Data deposited in or computed by PubChem | |
Molecular Formula |
C4H4O6-2 | |
Details | Computed by PubChem 2.2 (PubChem release 2021.10.14) | |
Source | PubChem | |
URL | https://pubchem.ncbi.nlm.nih.gov | |
Description | Data deposited in or computed by PubChem | |
DSSTOX Substance ID |
DTXSID901337655 | |
Record name | Tartrate | |
Source | EPA DSSTox | |
URL | https://comptox.epa.gov/dashboard/DTXSID901337655 | |
Description | DSSTox provides a high quality public chemistry resource for supporting improved predictive toxicology. | |
Molecular Weight |
148.07 g/mol | |
Details | Computed by PubChem 2.2 (PubChem release 2021.10.14) | |
Source | PubChem | |
URL | https://pubchem.ncbi.nlm.nih.gov | |
Description | Data deposited in or computed by PubChem | |
CAS No. |
3715-17-1, 64672-61-3 | |
Record name | Butanedioic acid, 2,3-dihydroxy- (2R,3R)-, ion(2-) | |
Source | ChemIDplus | |
URL | https://pubchem.ncbi.nlm.nih.gov/substance/?source=chemidplus&sourceid=0003715171 | |
Description | ChemIDplus is a free, web search system that provides access to the structure and nomenclature authority files used for the identification of chemical substances cited in National Library of Medicine (NLM) databases, including the TOXNET system. | |
Record name | Tartrate ion | |
Source | ChemIDplus | |
URL | https://pubchem.ncbi.nlm.nih.gov/substance/?source=chemidplus&sourceid=0064672613 | |
Description | ChemIDplus is a free, web search system that provides access to the structure and nomenclature authority files used for the identification of chemical substances cited in National Library of Medicine (NLM) databases, including the TOXNET system. | |
Record name | TARTRATE ION | |
Source | FDA Global Substance Registration System (GSRS) | |
URL | https://gsrs.ncats.nih.gov/ginas/app/beta/substances/GBG3U2YP5Y | |
Description | The FDA Global Substance Registration System (GSRS) enables the efficient and accurate exchange of information on what substances are in regulated products. Instead of relying on names, which vary across regulatory domains, countries, and regions, the GSRS knowledge base makes it possible for substances to be defined by standardized, scientific descriptions. | |
Explanation | Unless otherwise noted, the contents of the FDA website (www.fda.gov), both text and graphics, are not copyrighted. They are in the public domain and may be republished, reprinted and otherwise used freely by anyone without the need to obtain permission from FDA. Credit to the U.S. Food and Drug Administration as the source is appreciated but not required. | |
Synthesis routes and methods I
Procedure details
Synthesis routes and methods II
Procedure details
Synthesis routes and methods III
Procedure details
Retrosynthesis Analysis
AI-Powered Synthesis Planning: Our tool employs the Template_relevance Pistachio, Template_relevance Bkms_metabolic, Template_relevance Pistachio_ringbreaker, Template_relevance Reaxys, Template_relevance Reaxys_biocatalysis model, leveraging a vast database of chemical reactions to predict feasible synthetic routes.
One-Step Synthesis Focus: Specifically designed for one-step synthesis, it provides concise and direct routes for your target compounds, streamlining the synthesis process.
Accurate Predictions: Utilizing the extensive PISTACHIO, BKMS_METABOLIC, PISTACHIO_RINGBREAKER, REAXYS, REAXYS_BIOCATALYSIS database, our tool offers high-accuracy predictions, reflecting the latest in chemical research and data.
Strategy Settings
Precursor scoring | Relevance Heuristic |
---|---|
Min. plausibility | 0.01 |
Model | Template_relevance |
Template Set | Pistachio/Bkms_metabolic/Pistachio_ringbreaker/Reaxys/Reaxys_biocatalysis |
Top-N result to add to graph | 6 |
Feasible Synthetic Routes
Disclaimer and Information on In-Vitro Research Products
Please be aware that all articles and product information presented on BenchChem are intended solely for informational purposes. The products available for purchase on BenchChem are specifically designed for in-vitro studies, which are conducted outside of living organisms. In-vitro studies, derived from the Latin term "in glass," involve experiments performed in controlled laboratory settings using cells or tissues. It is important to note that these products are not categorized as medicines or drugs, and they have not received approval from the FDA for the prevention, treatment, or cure of any medical condition, ailment, or disease. We must emphasize that any form of bodily introduction of these products into humans or animals is strictly prohibited by law. It is essential to adhere to these guidelines to ensure compliance with legal and ethical standards in research and experimentation.