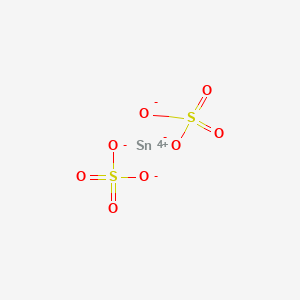
Tin sulfate
- Click on QUICK INQUIRY to receive a quote from our team of experts.
- With the quality product at a COMPETITIVE price, you can focus more on your research.
Overview
Description
Tin sulfate, also known as stannous sulfate, is a chemical compound with the formula SnSO₄. It is a white or yellowish crystalline solid that is highly soluble in water. This compound is known for its deliquescent properties, meaning it can absorb moisture from the air and dissolve in it. This compound is primarily used in electroplating, as a reducing agent, and in various industrial applications.
Preparation Methods
Synthetic Routes and Reaction Conditions: Tin sulfate can be synthesized through several methods. One common laboratory method involves the reaction between metallic tin and sulfuric acid: [ \text{Sn} + \text{H}_2\text{SO}_4 \rightarrow \text{SnSO}_4 + \text{H}_2 ]
Another method involves the displacement reaction between metallic tin and copper(II) sulfate: [ \text{Sn} + \text{CuSO}_4 \rightarrow \text{Cu} + \text{SnSO}_4 ]
Industrial Production Methods: In industrial settings, this compound is often produced by reacting tin with sulfuric acid under controlled conditions. The reaction is typically carried out at elevated temperatures to ensure complete conversion of tin to this compound. The resulting product is then purified through crystallization or other separation techniques.
Types of Reactions:
-
Oxidation: this compound can undergo oxidation to form tin(IV) sulfate. [ 2 \text{SnSO}_4 + \text{O}_2 \rightarrow 2 \text{Sn(SO}_4)_2 ]
-
Reduction: this compound acts as a reducing agent in various chemical reactions. [ \text{SnSO}_4 + 2 \text{H}_2 \rightarrow \text{Sn} + \text{H}_2\text{SO}_4 ]
-
Substitution: this compound can participate in substitution reactions with other metal salts. [ \text{SnSO}_4 + \text{Cu} \rightarrow \text{CuSO}_4 + \text{Sn} ]
Common Reagents and Conditions:
Oxidizing Agents: Oxygen, hydrogen peroxide.
Reducing Agents: Hydrogen gas, other metals.
Reaction Conditions: Elevated temperatures, controlled atmospheres.
Major Products Formed:
Oxidation: Tin(IV) sulfate.
Reduction: Metallic tin.
Substitution: Copper sulfate, other metal sulfates.
Scientific Research Applications
Tin sulfate has a wide range of applications in scientific research and industry:
Chemistry: Used as a reducing agent in organic synthesis and as a catalyst in various chemical reactions.
Biology: Employed in the preparation of biological samples for electron microscopy.
Medicine: Investigated for its potential use in medical imaging and as a component in certain pharmaceuticals.
Mechanism of Action
Tin sulfate acts primarily as a reducing agent, donating electrons to other compounds in chemical reactions. This property makes it useful in various industrial processes, such as electroplating and organic synthesis. In lead-acid batteries, this compound improves battery performance by altering the structure of the lead sulfate layer on the battery plates, enhancing corrosion resistance and charge acceptance .
Comparison with Similar Compounds
- Tin(II) chloride (SnCl₂)
- Tin(II) bromide (SnBr₂)
- Tin(II) iodide (SnI₂)
- Lead(II) sulfate (PbSO₄)
Comparison:
- Tin(II) chloride, bromide, and iodide: These compounds are similar to tin sulfate in that they contain tin in the +2 oxidation state. they differ in their anionic components and specific applications. For example, tin(II) chloride is commonly used as a reducing agent and in the preparation of other tin compounds.
- Lead(II) sulfate: This compound is similar to this compound in its sulfate anion but differs in its cation. Lead(II) sulfate is primarily used in lead-acid batteries and has different chemical properties and applications compared to this compound .
This compound stands out due to its unique combination of properties, including its solubility, reducing capabilities, and versatility in various applications.
Properties
CAS No. |
10031-62-6 |
---|---|
Molecular Formula |
H2O4SSn |
Molecular Weight |
216.79 g/mol |
IUPAC Name |
tin(4+);disulfate |
InChI |
InChI=1S/H2O4S.Sn/c1-5(2,3)4;/h(H2,1,2,3,4); |
InChI Key |
CICKVIRTJQTMFM-UHFFFAOYSA-N |
SMILES |
[O-]S(=O)(=O)[O-].[O-]S(=O)(=O)[O-].[Sn+4] |
Canonical SMILES |
OS(=O)(=O)O.[Sn] |
19307-28-9 | |
Related CAS |
19307-28-9 |
Synonyms |
stannous sulfate stannous sulfate, 113-labeled 1-2Sn salt stannous sulfate, 113-labeled Sn salt stannous sulfate, 117-labeled Sn salt stannous sulfate, 119-labeled Sn salt tin sulfate |
Origin of Product |
United States |
Synthesis routes and methods
Procedure details
Disclaimer and Information on In-Vitro Research Products
Please be aware that all articles and product information presented on BenchChem are intended solely for informational purposes. The products available for purchase on BenchChem are specifically designed for in-vitro studies, which are conducted outside of living organisms. In-vitro studies, derived from the Latin term "in glass," involve experiments performed in controlled laboratory settings using cells or tissues. It is important to note that these products are not categorized as medicines or drugs, and they have not received approval from the FDA for the prevention, treatment, or cure of any medical condition, ailment, or disease. We must emphasize that any form of bodily introduction of these products into humans or animals is strictly prohibited by law. It is essential to adhere to these guidelines to ensure compliance with legal and ethical standards in research and experimentation.