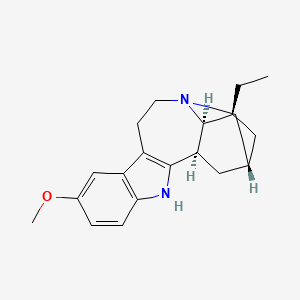
Ibogaine
Overview
Description
Ibogaine is a naturally occurring psychoactive indole alkaloid found in several plant species, most notably in the root bark of the African shrub Tabernanthe iboga. It has been traditionally used in West African spiritual ceremonies and rituals. This compound is known for its potential therapeutic effects, particularly in the treatment of substance use disorders, due to its ability to reduce withdrawal symptoms and cravings .
Mechanism of Action
Target of Action
Ibogaine, an indole alkaloid derived from the African shrub Tabernanthe iboga, has a complex pharmacology, affecting many different neurotransmitter systems simultaneously . It primarily targets the opioid system, crucial in addiction and pain regulation . It is active on sigma-2 and multiple opioid receptor sites . It also interacts with acetylcholine, dopamine, and serotonin systems .
Mode of Action
This compound interacts with its targets by binding to and modulating the activity of these receptors, altering the release and reuptake of neurotransmitters . This modulation is believed to contribute to the reduction of withdrawal symptoms and cravings observed in individuals undergoing this compound therapy for opioid addiction .
Biochemical Pathways
This compound’s activity is achieved through different types of receptors, but also rapid depletion of ATP, that is followed by the induction of energy-related enzymes, as well as rise of cellular reactive oxygen species (ROS) and antioxidant enzymes activity . It is also known to modulate intracellular signaling linked to opioid receptors .
Pharmacokinetics
When given orally, this compound is absorbed rapidly and undergoes extensive first-pass metabolism to 12-hydroxyibogamine (northis compound) by cytochrome P450 2D6 (CYP2D6) in the gut wall and liver . Plasma protein binding is in the range of 65–70% and consistent with its lipophilic nature, this compound accumulates in fat . The plasma half-life of this compound is in the range of 2–6 hours depending on CYP2D6 genotype, while the metabolite northis compound is eliminated with a half-life of 24–30 hours .
Result of Action
The molecular and cellular effects of this compound’s action include an increased amount of the neurotransmitter in the synaptic cleft and prolongs its effect on target cells . It also leads to potential synergies by modulating multiple receptor systems .
Action Environment
The setting in which this compound is consumed involves aspects of the environment in which the person is located while using the substance, and it includes factors such as culture, context of use, environmental stimuli (light, music, decoration) and presence (or absence) of other people . The use of controlled settings, supported by trained professionals and equipment allowing for rigorous medical, psychiatric, and cardiac monitoring, are essential to promote the safety of patients receiving this compound .
Biochemical Analysis
Biochemical Properties
Ibogaine plays a significant role in various biochemical reactions. It interacts with several enzymes, proteins, and other biomolecules. One of the primary enzymes involved in its metabolism is cytochrome P450 2D6 (CYP2D6), which converts this compound into its active metabolite, northis compound . This compound also interacts with mu-opioid receptors, serotonin transporters, and N-methyl-D-aspartate (NMDA) receptors, influencing neurotransmitter systems and receptor signaling .
Cellular Effects
This compound affects various cell types and cellular processes. It influences cell function by modulating cell signaling pathways, gene expression, and cellular metabolism. For example, this compound has been shown to impact the expression of genes involved in neurotransmitter systems, such as dopamine and serotonin pathways . Additionally, this compound’s interaction with NMDA receptors affects calcium ion influx, which can influence cellular metabolism and signaling .
Molecular Mechanism
At the molecular level, this compound exerts its effects through several mechanisms. It binds to mu-opioid receptors, acting as an agonist, and modulates serotonin transporters, enhancing serotonin levels in the synaptic cleft . This compound also inhibits NMDA receptors, reducing excitotoxicity and promoting neuroprotection . These interactions lead to changes in gene expression, particularly in genes related to neurotransmitter systems and receptor signaling .
Temporal Effects in Laboratory Settings
In laboratory settings, the effects of this compound change over time. This compound is rapidly absorbed and metabolized into northis compound, which has a longer half-life and sustained effects . Studies have shown that this compound’s effects on cellular function, such as neurotransmitter release and receptor signaling, can persist for several hours to days . The stability and degradation of this compound and its metabolites can influence the duration and intensity of its effects .
Dosage Effects in Animal Models
The effects of this compound vary with different dosages in animal models. At lower doses, this compound has been shown to reduce drug-seeking behavior and withdrawal symptoms in models of substance use disorders . At higher doses, this compound can cause toxic effects, such as neurotoxicity and cardiotoxicity . These adverse effects highlight the importance of careful dosage management in therapeutic applications .
Metabolic Pathways
This compound is involved in several metabolic pathways. It is primarily metabolized by cytochrome P450 2D6 (CYP2D6) into northis compound . This metabolic conversion is crucial for this compound’s therapeutic effects, as northis compound has a longer half-life and sustained activity . Additionally, this compound’s metabolism can influence metabolic flux and metabolite levels, affecting its overall pharmacokinetics and pharmacodynamics .
Transport and Distribution
This compound is transported and distributed within cells and tissues through various mechanisms. It is lipophilic, allowing it to accumulate in fatty tissues . This compound also interacts with plasma proteins, which can influence its distribution and bioavailability . Transporters and binding proteins, such as serotonin transporters, play a role in this compound’s localization and accumulation within specific tissues .
Subcellular Localization
The subcellular localization of this compound affects its activity and function. This compound can be found in various cellular compartments, including the cytoplasm and mitochondria . Post-translational modifications and targeting signals may direct this compound to specific organelles, influencing its interactions with biomolecules and its overall efficacy . Understanding the subcellular localization of this compound is essential for elucidating its mechanisms of action and therapeutic potential .
Preparation Methods
Synthetic Routes and Reaction Conditions: Ibogaine can be synthesized through several methods. One common approach involves the semi-synthesis from voacangine, another plant alkaloid. The total synthesis of this compound was first described in 1956. The process typically involves multiple steps, including the formation of key intermediates and the use of specific reagents and catalysts to achieve the desired chemical transformations .
Industrial Production Methods: Industrial production of this compound often relies on the extraction from the root bark of Tabernanthe iboga. The extraction process involves the use of solvents to isolate the alkaloid, followed by purification steps to obtain this compound in its pure form. Semi-synthetic methods using voacangine as a precursor are also employed in industrial settings .
Chemical Reactions Analysis
Types of Reactions: Ibogaine undergoes various chemical reactions, including oxidation, reduction, and substitution reactions. These reactions are essential for modifying the structure of this compound and exploring its derivatives for potential therapeutic applications .
Common Reagents and Conditions:
Oxidation: Common oxidizing agents such as potassium permanganate or chromium trioxide can be used to oxidize this compound, leading to the formation of different oxidation products.
Reduction: Reducing agents like lithium aluminum hydride or sodium borohydride are employed to reduce this compound, resulting in the formation of reduced derivatives.
Substitution: Substitution reactions often involve the use of halogens or other electrophiles to introduce new functional groups into the this compound molecule
Major Products: The major products formed from these reactions include various this compound derivatives, which are studied for their potential pharmacological properties. These derivatives can exhibit different levels of psychoactivity and therapeutic potential .
Scientific Research Applications
Ibogaine has garnered significant attention in scientific research due to its diverse applications:
Chemistry: this compound and its derivatives are studied for their unique chemical structures and potential as lead compounds for drug development.
Biology: Research focuses on the biological effects of this compound, including its impact on neurotransmitter systems and neuroplasticity.
Medicine: this compound is primarily investigated for its potential in treating substance use disorders, particularly opioid addiction. It is also explored for its effects on mood disorders and neurodegenerative diseases.
Industry: this compound’s unique properties make it a subject of interest in the pharmaceutical industry for developing new therapeutic agents
Comparison with Similar Compounds
Ibogaine is part of a family of structurally similar alkaloids known as iboga alkaloids. Some of the similar compounds include:
Northis compound: A metabolite of this compound with similar anti-addictive properties but reduced psychoactivity.
18-Methoxycoronaridine (18-MC): A synthetic derivative of this compound designed to lack psychedelic effects while retaining anti-addictive properties.
Tabernanthalog (TBG): A non-hallucinogenic analogue of this compound developed to treat addiction and depression with fewer side effects .
This compound’s uniqueness lies in its broad spectrum of activity across multiple neurotransmitter systems and its potential to induce long-lasting changes in brain function, making it a promising candidate for treating various neurological and psychiatric disorders.
Properties
CAS No. |
83-74-9 |
---|---|
Molecular Formula |
C20H26N2O |
Molecular Weight |
310.4 g/mol |
IUPAC Name |
(1R,15S)-17-ethyl-7-methoxy-3,13-diazapentacyclo[13.3.1.02,10.04,9.013,18]nonadeca-2(10),4(9),5,7-tetraene |
InChI |
InChI=1S/C20H26N2O/c1-3-13-8-12-9-17-19-15(6-7-22(11-12)20(13)17)16-10-14(23-2)4-5-18(16)21-19/h4-5,10,12-13,17,20-21H,3,6-9,11H2,1-2H3/t12-,13?,17-,20?/m0/s1 |
InChI Key |
HSIBGVUMFOSJPD-WBPAKMTCSA-N |
Isomeric SMILES |
CCC1C[C@H]2C[C@@H]3C1N(C2)CCC4=C3NC5=C4C=C(C=C5)OC |
SMILES |
CCC1CC2CC3C1N(C2)CCC4=C3NC5=C4C=C(C=C5)OC |
Canonical SMILES |
CCC1CC2CC3C1N(C2)CCC4=C3NC5=C4C=C(C=C5)OC |
melting_point |
148 °C Crystals from ethyl acetate. mp: 132 °C /Ibopamine hydrochloride/ |
83-74-9 | |
Pictograms |
Irritant |
shelf_life |
Stable under recommended storage conditions. /Ibogaine hydrochloride/ |
solubility |
Soluble in chloroform |
Synonyms |
12 Methoxyibogamine 12-Methoxyibogamine Endabuse Ibogaine NIH 10567 NIH-10567 NIH10567 |
vapor_pressure |
3.03X10-8 mm Hg at 25 °C (est) |
Origin of Product |
United States |
Retrosynthesis Analysis
AI-Powered Synthesis Planning: Our tool employs the Template_relevance Pistachio, Template_relevance Bkms_metabolic, Template_relevance Pistachio_ringbreaker, Template_relevance Reaxys, Template_relevance Reaxys_biocatalysis model, leveraging a vast database of chemical reactions to predict feasible synthetic routes.
One-Step Synthesis Focus: Specifically designed for one-step synthesis, it provides concise and direct routes for your target compounds, streamlining the synthesis process.
Accurate Predictions: Utilizing the extensive PISTACHIO, BKMS_METABOLIC, PISTACHIO_RINGBREAKER, REAXYS, REAXYS_BIOCATALYSIS database, our tool offers high-accuracy predictions, reflecting the latest in chemical research and data.
Strategy Settings
Precursor scoring | Relevance Heuristic |
---|---|
Min. plausibility | 0.01 |
Model | Template_relevance |
Template Set | Pistachio/Bkms_metabolic/Pistachio_ringbreaker/Reaxys/Reaxys_biocatalysis |
Top-N result to add to graph | 6 |
Feasible Synthetic Routes
Q1: How does ibogaine exert its effects within the body?
A1: this compound interacts with various neurotransmitter systems, exhibiting micromolar affinity for N-methyl-D-aspartate (NMDA), κ-opioid, μ-opioid, and sigma-2 receptors []. It also interacts with acetylcholine, serotonin, and dopamine systems, influencing the expression of proteins like substance P, brain-derived neurotrophic factor (BDNF), c-fos, and egr-1 []. This compound's primary metabolite, northis compound, also demonstrates biological activity, significantly contributing to this compound's overall pharmacological profile [].
Q2: What are the key differences in the pharmacological profiles of this compound and northis compound?
A2: While both this compound and northis compound exhibit anti-addictive properties in animal models, they differ in potency and side-effect profile. Notably, northis compound is a more potent indirect serotonin agonist than this compound, demonstrating a 10-fold higher potency in increasing extracellular serotonin levels in the nucleus accumbens of rats []. Additionally, northis compound appears to have a reduced propensity to induce adverse effects like tremors compared to this compound, suggesting it might be a safer alternative for medication development [].
Q3: What role does glial cell line-derived neurotrophic factor (GDNF) play in this compound's actions against alcohol consumption?
A4: Research suggests that GDNF in the ventral tegmental area (VTA) is crucial in mediating this compound's effects on reducing alcohol consumption []. this compound has been shown to increase GDNF expression in the VTA, and this increase is associated with a reduction in ethanol self-administration in rodents [, ]. The effect of this compound on ethanol consumption can be mimicked by injecting GDNF directly into the VTA and can be attenuated by injecting anti-GDNF antibodies, further supporting the critical role of GDNF in this compound's actions [].
Q4: How is this compound metabolized in the body?
A5: this compound is primarily metabolized by the cytochrome P450 enzyme CYP2D6 to its main metabolite, northis compound [, , ]. Northis compound exhibits clinically relevant concentrations for an extended period, even after this compound is cleared from the system []. This prolonged presence of northis compound is likely due to its slow release from fat tissue, where both this compound and northis compound are sequestered [].
Q5: What is the significance of CYP2D6 polymorphisms in this compound metabolism?
A6: Genetic variations in the CYP2D6 enzyme can significantly influence the pharmacokinetic profiles of both this compound and northis compound [, ]. Patients identified as CYP2D6 poor metabolizers exhibit a 3-fold higher blood concentration of this compound and a 4-fold lower concentration of northis compound compared to extensive metabolizers []. This suggests that individuals with different CYP2D6 metabolic phenotypes may exhibit varied sensitivity to this compound and northis compound, potentially influencing therapeutic outcomes and safety profiles [].
Q6: What are the implications of this compound and northis compound sequestration in fat tissue?
A7: Both this compound and its active metabolite, northis compound, are sequestered in fat tissue [, ]. This sequestration contributes to their complex pharmacokinetic profiles, characterized by a long elimination half-life and prolonged presence in the body even after a single dose [, ]. The slow release of this compound and northis compound from fat stores could contribute to the long-lasting effects observed after a single administration [].
Q7: Are there alternative compounds being explored that might offer a safer profile than this compound?
A11: The development of safer analogs of this compound with reduced toxicity is an active area of research []. One such compound, 18-methoxycoronaridine (18-MC), has shown promise in preclinical studies. 18-MC, a synthetic derivative of this compound, exhibits similar anti-addictive properties in rodent models without some of this compound's adverse side effects, such as tremors and cerebellar damage []. Its lower affinity for NMDA and sigma-2 receptors, compared to this compound, is thought to contribute to its improved safety profile [].
Q8: What are the critical areas for future research on this compound?
A12: Future research should prioritize well-controlled clinical trials to assess the long-term safety and efficacy of this compound in treating substance use disorders [, , ]. Exploring personalized medicine approaches based on CYP2D6 genotyping could potentially optimize dosing and minimize adverse effects []. Further investigation into the mechanisms of action, particularly the role of different receptors and signaling pathways, is crucial for understanding this compound's complex pharmacology and developing safer and more effective therapies [].
Disclaimer and Information on In-Vitro Research Products
Please be aware that all articles and product information presented on BenchChem are intended solely for informational purposes. The products available for purchase on BenchChem are specifically designed for in-vitro studies, which are conducted outside of living organisms. In-vitro studies, derived from the Latin term "in glass," involve experiments performed in controlled laboratory settings using cells or tissues. It is important to note that these products are not categorized as medicines or drugs, and they have not received approval from the FDA for the prevention, treatment, or cure of any medical condition, ailment, or disease. We must emphasize that any form of bodily introduction of these products into humans or animals is strictly prohibited by law. It is essential to adhere to these guidelines to ensure compliance with legal and ethical standards in research and experimentation.