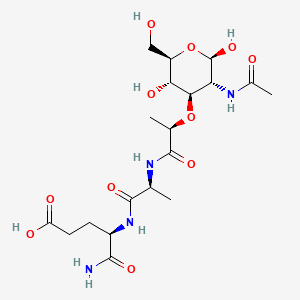
Muramyl Dipeptide
- Click on QUICK INQUIRY to receive a quote from our team of experts.
- With the quality product at a COMPETITIVE price, you can focus more on your research.
Overview
Description
Muramyl dipeptide is a component of bacterial peptidoglycan, which is a recognition structure or activator for nucleotide-binding oligomerization domain 2 (NOD2) protein. It is a constituent of both Gram-positive and Gram-negative bacteria, composed of N-acetylmuramic acid linked by its lactic acid moiety to the N-terminus of an L-alanine D-isoglutamine dipeptide . This compound is recognized by the immune system as a pathogen-associated molecular pattern and activates the NALP3 inflammasome, leading to cytokine activation, particularly interleukin-1α and interleukin-1β .
Preparation Methods
Synthetic Routes and Reaction Conditions
Muramyl dipeptide can be synthesized through various methods. One common synthetic route involves the coupling of N-acetylmuramic acid with L-alanine and D-isoglutamine using peptide coupling reagents such as dicyclohexylcarbodiimide (DCC) and N-hydroxysuccinimide (NHS) . The reaction typically occurs in an organic solvent like dimethylformamide (DMF) under mild conditions.
Industrial Production Methods
Industrial production of this compound often involves fermentation processes using genetically engineered bacteria that overproduce the compound. The bacteria are cultured in large bioreactors, and the compound is extracted and purified using chromatographic techniques .
Chemical Reactions Analysis
Types of Reactions
Muramyl dipeptide undergoes various chemical reactions, including:
Oxidation: this compound can be oxidized using oxidizing agents like hydrogen peroxide or potassium permanganate.
Reduction: Reduction reactions can be carried out using reducing agents such as sodium borohydride.
Substitution: Substitution reactions can occur at the amino or hydroxyl groups using reagents like alkyl halides or acyl chlorides.
Common Reagents and Conditions
Oxidation: Hydrogen peroxide in aqueous solution at room temperature.
Reduction: Sodium borohydride in methanol at low temperatures.
Substitution: Alkyl halides or acyl chlorides in the presence of a base like triethylamine.
Major Products Formed
Oxidation: Oxidized derivatives of this compound.
Reduction: Reduced forms of this compound.
Substitution: Substituted this compound derivatives.
Scientific Research Applications
Muramyl dipeptide has a wide range of scientific research applications:
Chemistry: Used as a model compound to study peptidoglycan structure and function.
Biology: Investigated for its role in immune system activation and pathogen recognition.
Mechanism of Action
Muramyl dipeptide exerts its effects by binding to the NOD2 receptor, a cytoplasmic receptor involved in the host innate immune system defense. Upon binding, it activates the NALP3 inflammasome, leading to the production of proinflammatory cytokines such as interleukin-1α and interleukin-1β . This activation triggers a cascade of immune responses, enhancing the body’s ability to recognize and respond to bacterial infections .
Comparison with Similar Compounds
Similar Compounds
Mifamurtide: A synthetic analogue of muramyl dipeptide used in the treatment of osteosarcoma.
Desmuramyl peptides: Modified analogues of this compound with improved immunostimulant properties.
N-glycolyl this compound: A derivative with enhanced immune response capabilities.
Uniqueness
This compound is unique due to its ability to activate the NOD2 receptor and the NALP3 inflammasome, leading to a robust immune response. Its structural components, including N-acetylmuramic acid and the L-alanine D-isoglutamine dipeptide, are critical for its biological activity .
Properties
CAS No. |
87420-48-2 |
---|---|
Molecular Formula |
C19H32N4O11 |
Molecular Weight |
492.5 g/mol |
IUPAC Name |
(4R)-4-[[(2S)-2-[[(2R)-2-[(2R,3R,4R,5S,6R)-3-acetamido-2,5-dihydroxy-6-(hydroxymethyl)oxan-4-yl]oxypropanoyl]amino]propanoyl]amino]-5-amino-5-oxopentanoic acid |
InChI |
InChI=1S/C19H32N4O11/c1-7(17(30)23-10(16(20)29)4-5-12(26)27)21-18(31)8(2)33-15-13(22-9(3)25)19(32)34-11(6-24)14(15)28/h7-8,10-11,13-15,19,24,28,32H,4-6H2,1-3H3,(H2,20,29)(H,21,31)(H,22,25)(H,23,30)(H,26,27)/t7-,8+,10+,11+,13+,14+,15+,19+/m0/s1 |
InChI Key |
BSOQXXWZTUDTEL-ZUYCGGNHSA-N |
SMILES |
CC(C(=O)NC(CCC(=O)O)C(=O)N)NC(=O)C(C)OC1C(C(OC(C1O)CO)O)NC(=O)C |
Isomeric SMILES |
C[C@@H](C(=O)N[C@H](CCC(=O)O)C(=O)N)NC(=O)[C@@H](C)O[C@@H]1[C@H]([C@@H](O[C@@H]([C@H]1O)CO)O)NC(=O)C |
Canonical SMILES |
CC(C(=O)NC(CCC(=O)O)C(=O)N)NC(=O)C(C)OC1C(C(OC(C1O)CO)O)NC(=O)C |
Key on ui other cas no. |
53678-77-6 |
Synonyms |
Acetylmuramyl Alanyl Isoglutamine Acetylmuramyl-Alanyl-Isoglutamine Alanyl Isoglutamine, Acetylmuramyl Dipeptide, Muramyl Isoglutamine, Acetylmuramyl Alanyl Mur NAc L Ala D isoGln Mur-NAc-L-Ala-D-isoGln Muramyl Dipeptide N Acetyl Muramyl L Alanyl D Glutamic alpha Amide N Acetylmuramyl L Alanyl D Isoglutamine N-Acetyl-Muramyl-L-Alanyl-D-Glutamic-alpha-Amide N-Acetylmuramyl-L-Alanyl-D-Isoglutamine |
Origin of Product |
United States |
Retrosynthesis Analysis
AI-Powered Synthesis Planning: Our tool employs the Template_relevance Pistachio, Template_relevance Bkms_metabolic, Template_relevance Pistachio_ringbreaker, Template_relevance Reaxys, Template_relevance Reaxys_biocatalysis model, leveraging a vast database of chemical reactions to predict feasible synthetic routes.
One-Step Synthesis Focus: Specifically designed for one-step synthesis, it provides concise and direct routes for your target compounds, streamlining the synthesis process.
Accurate Predictions: Utilizing the extensive PISTACHIO, BKMS_METABOLIC, PISTACHIO_RINGBREAKER, REAXYS, REAXYS_BIOCATALYSIS database, our tool offers high-accuracy predictions, reflecting the latest in chemical research and data.
Strategy Settings
Precursor scoring | Relevance Heuristic |
---|---|
Min. plausibility | 0.01 |
Model | Template_relevance |
Template Set | Pistachio/Bkms_metabolic/Pistachio_ringbreaker/Reaxys/Reaxys_biocatalysis |
Top-N result to add to graph | 6 |
Feasible Synthetic Routes
Disclaimer and Information on In-Vitro Research Products
Please be aware that all articles and product information presented on BenchChem are intended solely for informational purposes. The products available for purchase on BenchChem are specifically designed for in-vitro studies, which are conducted outside of living organisms. In-vitro studies, derived from the Latin term "in glass," involve experiments performed in controlled laboratory settings using cells or tissues. It is important to note that these products are not categorized as medicines or drugs, and they have not received approval from the FDA for the prevention, treatment, or cure of any medical condition, ailment, or disease. We must emphasize that any form of bodily introduction of these products into humans or animals is strictly prohibited by law. It is essential to adhere to these guidelines to ensure compliance with legal and ethical standards in research and experimentation.