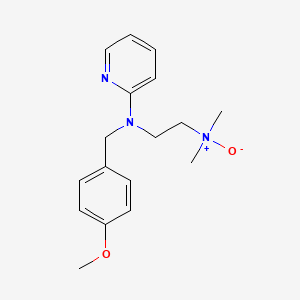
Pyrilamine N-oxide
Overview
Description
Pyrilamine N-oxide is a primary oxidative metabolite of pyrilamine, a first-generation antihistamine used for its H1 receptor antagonism. It is formed via N-oxidation of the dimethylaminoethyl group in pyrilamine, a reaction catalyzed by hepatic cytochrome P450 enzymes or microbial systems . Unlike the parent compound, this compound exhibits increased polarity, influencing its pharmacokinetic behavior, including reduced tissue accumulation and altered excretion pathways . Studies in rats indicate that this compound is a minor metabolite compared to O-demethyl-pyrilamine glucuronide, the major urinary metabolite .
Preparation Methods
Oxidation Methods Using Peracids
m-Chloroperoxybenzoic Acid (mCPBA) as the Oxidizing Agent
The most widely reported method for synthesizing pyridine N-oxides, including pyrilamine N-oxide, involves the use of mCPBA in dichloromethane (DCM). A patented process outlines the following steps:
-
Reaction Setup : Pyridine derivatives (e.g., 3-chloropyridine, 4-methylpyridine) are dissolved in DCM at 0–5°C.
-
Oxidation : mCPBA is added gradually to the cooled solution, followed by stirring at 20–25°C for 24 hours.
-
Workup : The reaction mixture is concentrated under reduced pressure, resuspended in water, and adjusted to pH 4–5 to precipitate byproducts (e.g., m-chlorobenzoic acid).
-
Purification : Filtration and drying yield the N-oxide product with purities exceeding 95% and yields of 90–95% .
Reaction Equation :
Table 1: Representative Reaction Conditions for mCPBA-Mediated N-Oxidation
Pyridine Derivative | mCPBA Equivalents | Solvent (DCM) Volume | Yield (%) | Purity (%) |
---|---|---|---|---|
3-Chloropyridine | 1.5 | 2.44 mL/g | 95 | 95 |
4-Methylpyridine | 1.5 | 2.64 mL/g | 93 | 95 |
2,6-Dichloropyridine | 1.5 | 2.91 mL/g | 92 | 94 |
The high efficiency of mCPBA is attributed to its electrophilic peroxy group, which facilitates nucleophilic attack by the pyridine nitrogen. However, the exothermic nature of the reaction necessitates precise temperature control to avoid decomposition .
Alternative Synthetic Routes
Enamine Condensation and Cyclization
A European patent discloses a multistep route for functionalized pyridine N-oxides, applicable to pyrilamine analogs:
-
Enamine Formation : Condensation of acetyl chloride derivatives with alkyl vinyl ethers.
-
Cyclization : Reaction with amine nucleophiles under reflux to form the pyridine core.
-
Oxidation : Subsequent treatment with peracids to yield the N-oxide.
While this method allows for structural diversification, it involves complex intermediates and longer reaction times compared to direct oxidation .
Microreactor-Based Synthesis
Recent advancements in flow chemistry have enabled safer and more efficient N-oxidation. A microreactor study demonstrated the following advantages:
-
Enhanced Heat Management : The high surface-to-volume ratio of microreactors mitigates thermal runaway risks.
-
Reduced Reaction Time : Complete oxidation of pyridine derivatives was achieved in 1–2 hours, versus 24 hours in batch processes .
-
Scalability : Continuous flow systems allow for gram-scale production without compromising yield or purity.
Table 2: Comparison of Batch vs. Microreactor N-Oxidation
Parameter | Batch Process | Microreactor |
---|---|---|
Reaction Time (h) | 24 | 1.5 |
Temperature Control | Challenging | Precise |
Yield (%) | 90–95 | 88–93 |
Purification and Analytical Validation
Isolation Techniques
Post-reaction workup typically involves:
-
pH Adjustment : Acidification to pH 4–5 precipitates byproducts, enabling facile filtration .
-
Solvent Removal : Rotary evaporation under reduced pressure isolates the crude product.
-
Drying : Diaphragm pumps remove residual solvents, yielding crystalline or powdered N-oxide .
Analytical Methods
-
Liquid Chromatography-Mass Spectrometry (LC-MS) : Confirms molecular ion peaks (e.g., m/z 125.9 for 4-methoxypyridine N-oxide) .
-
Nuclear Magnetic Resonance (NMR) : Validates structural integrity through characteristic shifts (e.g., δ 8.2–8.5 ppm for N-oxide protons) .
Applications and Pharmacological Significance
This compound’s role in drug metabolism has been elucidated in rodent studies, where it accounts for >50% of hepatic pyrilamine clearance . Its formation is catalyzed by cytochrome P450 enzymes, though synthetic routes bypass enzymatic limitations for large-scale production. Future research may explore its potential as a standalone therapeutic agent or prodrug.
Chemical Reactions Analysis
Types of Reactions: Pyrilamine N-oxide undergoes various chemical reactions, including:
Oxidation: Further oxidation can lead to the formation of more oxidized derivatives.
Reduction: Reduction of the N-oxide group can regenerate the parent pyrilamine compound.
Substitution: The N-oxide group can participate in nucleophilic substitution reactions, where it acts as a leaving group.
Common Reagents and Conditions:
Oxidation: Peroxyacids, hydrogen peroxide, and sodium percarbonate are commonly used oxidizing agents.
Reduction: Reducing agents like zinc and acetic acid can be employed to reduce the N-oxide group.
Substitution: Nucleophiles such as amines and thiols can react with the N-oxide group under mild conditions.
Major Products:
Oxidation: More oxidized derivatives of pyrilamine.
Reduction: Regeneration of pyrilamine.
Substitution: Substituted pyrilamine derivatives with various functional groups.
Scientific Research Applications
Medicinal Chemistry
Pyrilamine N-oxide is studied for its potential effects on histamine receptors, functioning as an inverse agonist. This mechanism allows it to stabilize the inactive form of the histamine H1 receptor, thereby reducing allergic responses.
- Case Study: Research indicated that the pharmacokinetic properties of this compound differ from those of its parent compound, suggesting improved efficacy in treating allergic conditions due to altered absorption and metabolism pathways .
Organic Synthesis
In organic chemistry, this compound serves as a mild oxidizing agent in various reactions. Its ability to facilitate oxidation processes makes it valuable in synthesizing other bioactive compounds.
- Application Example: The compound has been utilized in the Upjohn oxidation process, demonstrating its effectiveness as an oxidant in synthetic organic chemistry .
Biological Studies
Studies have shown that this compound can affect cellular metabolism and membrane transport activities, influencing drug metabolism pathways.
- Research Findings: In rat liver studies, Pyrilamine was metabolized into two major metabolites, including this compound, highlighting its role in drug metabolism .
Analytical Techniques for Detection
Various analytical techniques are employed to identify and quantify this compound and its metabolites:
Toxicological Studies
The safety profile of this compound is crucial for its application in medicine. Toxicological assessments indicate that while it exhibits antihistaminic properties, further studies are necessary to fully understand its safety and efficacy.
- Research Insight: A comparative study on pulmonary accumulation showed that Pyrilamine accumulates significantly more than its N-oxide form in lung tissues, suggesting different pharmacokinetic behaviors that may impact therapeutic use .
Summary of Findings
This compound demonstrates significant potential across various fields:
- Medicinal Applications: As an antihistamine with modified pharmacokinetics.
- Organic Synthesis: As a reagent facilitating oxidation reactions.
- Biological Impact: Influencing drug metabolism and cellular responses.
Mechanism of Action
Pyrilamine N-oxide exerts its effects by targeting the histamine H1 receptor, similar to pyrilamine. It acts as an inverse agonist, binding to the receptor and stabilizing its inactive form, thereby reducing histamine-mediated allergic responses . The N-oxide group may influence the compound’s pharmacokinetics, potentially altering its absorption, distribution, metabolism, and excretion.
Comparison with Similar Compounds
Metabolic Pathways and Tissue Accumulation
Pyrilamine N-oxide is distinguished from other pyrilamine metabolites (e.g., O-demethyl, N-demethyl derivatives) by its formation pathway and tissue distribution. Key findings include:
- Lung Accumulation : In mice, pyrilamine accumulates in lung tissue at levels 10-fold higher than its N-oxide after perfusion, suggesting stronger tissue-specific binding of the parent compound .
- Excretion : In rats, this compound accounts for a smaller fraction of excreted metabolites compared to O-demethyl-pyrilamine glucuronide (29–38% urinary excretion of total dose) .
Table 1: Metabolic Pathways of Pyrilamine and Comparable N-Oxides
Pharmacokinetic Comparison with Other N-Oxides
Sorafenib N-oxide () and doxylamine N-oxide () serve as relevant comparators:
- Plasma and Brain Exposure: Co-administration of paracetamol with sorafenib increases the plasma AUC of sorafenib N-oxide by 22.6% (3.74 vs. 3.05 µg·h/mL) and brain AUC by 50.9%, suggesting enhanced bioavailability and central nervous system penetration .
- Elimination Half-Life : Pyrilamine’s plasma elimination half-life (1.5–2.3 hours) is dose-independent, whereas sorafenib N-oxide’s half-life is influenced by drug interactions .
Table 2: Pharmacokinetic Parameters
Structural and Analytical Characterization
- Mass Spectrometry : this compound and doxylamine N-oxide produce distinct [M+H]⁺ ions under thermospray mass spectrometry. Fragmentation patterns aid in differentiating N-oxides from dealkylated metabolites .
Toxicological and Mutagenic Profiles
- This contrasts with some N-oxides (e.g., nitrosamines), which are potent mutagens .
- Tissue Toxicity : Lower lung accumulation of this compound compared to the parent compound may reduce direct tissue toxicity .
Biological Activity
Pyrilamine N-oxide is a metabolite of pyrilamine, an antihistamine primarily used for allergic conditions. This compound has garnered attention due to its potential biological activities, particularly in the context of histamine receptor interactions and its metabolic pathways. The following sections detail the biological activity of this compound, supported by research findings and data tables.
This compound acts primarily as an H1 histamine receptor antagonist. Research indicates that it can modulate vascular responses through its interaction with nitric oxide (NO) pathways. In studies involving whole-body heating, pyrilamine significantly reduced cutaneous vascular conductance (CVC), suggesting its role in inhibiting vasodilation mediated by histamine .
2. Pharmacokinetics and Metabolism
The pharmacokinetic profile of this compound reveals that it is a major metabolite formed during the metabolism of pyrilamine. Studies show that after administration, pyrilamine undergoes extensive metabolism in the liver, resulting in the formation of this compound among other metabolites .
A comparative analysis of pulmonary accumulation between pyrilamine and this compound demonstrated that pyrilamine accumulates approximately ten times more than its N-oxide counterpart in mouse lungs . This differential accumulation may influence their respective biological effects.
3.1 Antihistaminic Activity
This compound exhibits antihistaminic properties by blocking H1 receptors, which can mitigate allergic responses. In controlled studies, it has been shown to effectively reduce symptoms associated with histamine release, such as vasodilation and increased vascular permeability.
3.2 Vascular Effects
The compound's effect on vascular dynamics was highlighted in a study where it was administered alongside l-NAME (a nitric oxide synthase inhibitor). Results indicated that this compound could significantly alter CVC when compared to control conditions, underscoring its role in vascular regulation during inflammatory responses .
4. Comparative Biological Activity Table
Parameter | Pyrilamine | This compound | Control |
---|---|---|---|
CVC during whole body heating (%) | 51 ± 3 | 33 ± 3 | 69 ± 3 |
Pulmonary accumulation (μM) | 40 | 4 | - |
Histamine-induced vasodilation (reduction %) | Significant | Moderate | None |
5. Toxicity and Safety Profiles
While this compound has demonstrated beneficial pharmacological effects, understanding its toxicity is crucial. Preliminary studies suggest low cytotoxicity at therapeutic levels, but further investigations are necessary to establish a comprehensive safety profile.
6. Conclusion
This compound represents a significant area of interest within pharmacology due to its antihistaminic properties and effects on vascular regulation. Its metabolism from pyrilamine highlights the importance of considering metabolites in drug efficacy and safety assessments. Continued research is essential to fully elucidate its biological mechanisms and potential therapeutic applications.
Q & A
Basic Research Questions
Q. How can the crystal structure of Pyrilamine N-oxide be experimentally determined?
- Methodology : Single-crystal X-ray diffraction (SC-XRD) is the gold standard for resolving molecular and supramolecular structures. Key parameters include unit cell dimensions (e.g., monoclinic systems with space group P2₁/c), bond lengths, and hydrogen-bonding networks. Data collection at low temperatures (e.g., 100 K) minimizes thermal motion artifacts. Software like OLEX2 or SHELX is used for refinement .
- Application : Structural data inform reactivity predictions, polymorphism studies, and interactions with biological targets.
Q. What analytical techniques are suitable for quantifying this compound in complex matrices?
- Methodology : Liquid chromatography-tandem mass spectrometry (LC-MS/MS) coupled with online solid-phase extraction (SPE) enables high-sensitivity quantification. Calibration curves (10–300 µg/kg range) with deuterated internal standards (e.g., trimethylamine-d9 N-oxide) improve precision. Weighted linear regression (r² > 0.99) and signal-to-noise ratios (S/N > 10) validate method robustness .
Q. How can researchers verify the purity and stability of this compound during synthesis?
- Methodology : Use nuclear magnetic resonance (NMR) spectroscopy (¹H/¹³C) to confirm chemical identity and assess impurities. Accelerated stability studies under varied pH, temperature, and light exposure monitor degradation. High-performance liquid chromatography (HPLC) with UV detection quantifies degradation products .
Advanced Research Questions
Q. What experimental designs are used to investigate the mutagenic potential of this compound?
- Methodology : Structure-activity relationship (SAR) fingerprint analysis evaluates substructure alerts (e.g., quindioxin-like motifs). Public/proprietary databases (e.g., Leadscope) are mined for mutagenicity data. In vitro Ames tests with Salmonella strains (TA98, TA100) under metabolic activation (S9 mix) validate predictions. Dose-response curves and statistical thresholds (e.g., twofold increase in revertants) differentiate mutagenic vs. non-mutagenic profiles .
Q. How do researchers resolve contradictions in transporter-mediated uptake mechanisms of this compound?
- Methodology : Compare cellular uptake in wild-type vs. transporter-knockout models (e.g., Oct1⁻/⁻ mice). Use hepatocellular carcinoma cells (HepG2, Huh7) and HEK293 lines overexpressing organic cation transporters (OCT1). Pharmacokinetic studies in rodents measure plasma and hepatic concentrations post-administration. Data normalization to protein content and statistical modeling (ANOVA) identify transporter-independent pathways .
Q. What strategies mitigate batch-to-batch variability in this compound bioactivity assays?
- Methodology : Implement strict quality control (QC) protocols:
- Chemical Characterization : Fourier-transform infrared (FTIR) spectroscopy and elemental analysis.
- Biological Replicates : Triplicate assays with positive/negative controls (e.g., sorafenib N-oxide for transporter studies).
- Data Normalization : Use Z-factor metrics to assess assay robustness and minimize false positives/negatives .
Q. How can meta-analysis frameworks evaluate epidemiological correlations between this compound exposure and clinical outcomes?
- Methodology : Aggregate data from cohort studies using PRISMA guidelines. Fixed/random-effects models calculate pooled hazard ratios (HRs). Dose-response analysis with restricted cubic splines identifies non-linear associations. Sensitivity analyses exclude outliers, and funnel plots assess publication bias. Tools like RevMan or R’s metafor package streamline statistical workflows .
Properties
IUPAC Name |
2-[(4-methoxyphenyl)methyl-pyridin-2-ylamino]-N,N-dimethylethanamine oxide | |
---|---|---|
Source | PubChem | |
URL | https://pubchem.ncbi.nlm.nih.gov | |
Description | Data deposited in or computed by PubChem | |
InChI |
InChI=1S/C17H23N3O2/c1-20(2,21)13-12-19(17-6-4-5-11-18-17)14-15-7-9-16(22-3)10-8-15/h4-11H,12-14H2,1-3H3 | |
Source | PubChem | |
URL | https://pubchem.ncbi.nlm.nih.gov | |
Description | Data deposited in or computed by PubChem | |
InChI Key |
IQDUZGGMNVZTAI-UHFFFAOYSA-N | |
Source | PubChem | |
URL | https://pubchem.ncbi.nlm.nih.gov | |
Description | Data deposited in or computed by PubChem | |
Canonical SMILES |
C[N+](C)(CCN(CC1=CC=C(C=C1)OC)C2=CC=CC=N2)[O-] | |
Source | PubChem | |
URL | https://pubchem.ncbi.nlm.nih.gov | |
Description | Data deposited in or computed by PubChem | |
Molecular Formula |
C17H23N3O2 | |
Source | PubChem | |
URL | https://pubchem.ncbi.nlm.nih.gov | |
Description | Data deposited in or computed by PubChem | |
DSSTOX Substance ID |
DTXSID70891604 | |
Record name | 2-{[(4-Methoxyphenyl)methyl](pyridin-2-yl)amino}-N,N-dimethylethan-1-amine N-oxide | |
Source | EPA DSSTox | |
URL | https://comptox.epa.gov/dashboard/DTXSID70891604 | |
Description | DSSTox provides a high quality public chemistry resource for supporting improved predictive toxicology. | |
Molecular Weight |
301.4 g/mol | |
Source | PubChem | |
URL | https://pubchem.ncbi.nlm.nih.gov | |
Description | Data deposited in or computed by PubChem | |
CAS No. |
98982-99-1 | |
Record name | Pyrilamine N-oxide | |
Source | ChemIDplus | |
URL | https://pubchem.ncbi.nlm.nih.gov/substance/?source=chemidplus&sourceid=0098982991 | |
Description | ChemIDplus is a free, web search system that provides access to the structure and nomenclature authority files used for the identification of chemical substances cited in National Library of Medicine (NLM) databases, including the TOXNET system. | |
Record name | 2-{[(4-Methoxyphenyl)methyl](pyridin-2-yl)amino}-N,N-dimethylethan-1-amine N-oxide | |
Source | EPA DSSTox | |
URL | https://comptox.epa.gov/dashboard/DTXSID70891604 | |
Description | DSSTox provides a high quality public chemistry resource for supporting improved predictive toxicology. | |
Retrosynthesis Analysis
AI-Powered Synthesis Planning: Our tool employs the Template_relevance Pistachio, Template_relevance Bkms_metabolic, Template_relevance Pistachio_ringbreaker, Template_relevance Reaxys, Template_relevance Reaxys_biocatalysis model, leveraging a vast database of chemical reactions to predict feasible synthetic routes.
One-Step Synthesis Focus: Specifically designed for one-step synthesis, it provides concise and direct routes for your target compounds, streamlining the synthesis process.
Accurate Predictions: Utilizing the extensive PISTACHIO, BKMS_METABOLIC, PISTACHIO_RINGBREAKER, REAXYS, REAXYS_BIOCATALYSIS database, our tool offers high-accuracy predictions, reflecting the latest in chemical research and data.
Strategy Settings
Precursor scoring | Relevance Heuristic |
---|---|
Min. plausibility | 0.01 |
Model | Template_relevance |
Template Set | Pistachio/Bkms_metabolic/Pistachio_ringbreaker/Reaxys/Reaxys_biocatalysis |
Top-N result to add to graph | 6 |
Feasible Synthetic Routes
Disclaimer and Information on In-Vitro Research Products
Please be aware that all articles and product information presented on BenchChem are intended solely for informational purposes. The products available for purchase on BenchChem are specifically designed for in-vitro studies, which are conducted outside of living organisms. In-vitro studies, derived from the Latin term "in glass," involve experiments performed in controlled laboratory settings using cells or tissues. It is important to note that these products are not categorized as medicines or drugs, and they have not received approval from the FDA for the prevention, treatment, or cure of any medical condition, ailment, or disease. We must emphasize that any form of bodily introduction of these products into humans or animals is strictly prohibited by law. It is essential to adhere to these guidelines to ensure compliance with legal and ethical standards in research and experimentation.