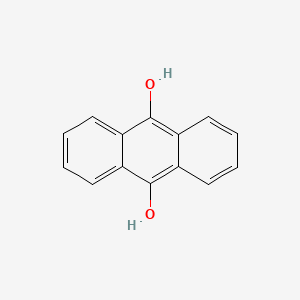
anthracene-9,10-diol
Overview
Description
anthracene-9,10-diol, also known as this compound, is an organic compound with the molecular formula C14H10O2. It is a derivative of anthracene, featuring hydroxyl groups at the 9 and 10 positions. This compound is known for its crystalline structure and is used in various scientific and industrial applications.
Mechanism of Action
Anthracene-9,10-diol, also known as 9,10-Dihydroxyanthracene or 9,10-Anthracenediol, is an organic compound with the formula C14H10O2
Target of Action
Anthracene derivatives have been known to interact with various biological targets, including enzymes and cellular structures .
Mode of Action
This compound is the hydroquinone form of 9,10-anthraquinone (AQ). It is formed when AQ is hydrogenated It’s worth noting that anthracene derivatives can undergo various chemical reactions, potentially leading to changes in their targets .
Biochemical Pathways
Anthracene derivatives have been known to interfere with various biochemical processes, potentially leading to downstream effects .
Pharmacokinetics
It is known that the compound is easily dissolved in alkaline solutions .
Result of Action
Anthracene derivatives can have various effects on cells, potentially leading to changes in cellular function .
Action Environment
Environmental factors can influence the action, efficacy, and stability of this compound. For instance, the compound’s solubility can be affected by the pH of the environment . Additionally, light exposure can influence the photophysics of anthracene derivatives .
Biochemical Analysis
Biochemical Properties
9,10-Anthracenediol plays a crucial role in biochemical reactions, particularly in the context of photophysics and as a sacrificial template in xerogels . It interacts with various biomolecules, including enzymes such as hydroxylase and dioxygenase, which facilitate its degradation and transformation
Cellular Effects
The effects of 9,10-Anthracenediol on cellular processes are multifaceted. It influences cell function by affecting cell signaling pathways, gene expression, and cellular metabolism. Studies have shown that 9,10-Anthracenediol can alter the photophysical properties of cells when sequestered within xerogels, leading to changes in electron and energy transfer processes
Molecular Mechanism
At the molecular level, 9,10-Anthracenediol exerts its effects through various binding interactions with biomolecules. It can act as a template for the formation of analyte-responsive sites in xerogels, influencing the distribution of microenvironments within the gel matrix . Additionally, 9,10-Anthracenediol’s interactions with enzymes such as hydroxylase and dioxygenase play a significant role in its degradation and transformation .
Temporal Effects in Laboratory Settings
In laboratory settings, the effects of 9,10-Anthracenediol change over time due to its stability and degradation properties. Studies have shown that the compound’s photophysical properties remain stable when dissolved in solution but exhibit greater variance when sequestered within xerogels . This temporal variability is essential for understanding the long-term effects of 9,10-Anthracenediol on cellular function in both in vitro and in vivo studies.
Dosage Effects in Animal Models
The effects of 9,10-Anthracenediol vary with different dosages in animal models. While specific studies on this compound’s dosage effects are limited, general principles of drug metabolism and toxicity in animal models suggest that higher doses may lead to adverse effects, including toxicity and changes in metabolic pathways . Understanding these dosage effects is crucial for determining safe and effective applications of 9,10-Anthracenediol in biomedical research.
Metabolic Pathways
9,10-Anthracenediol is involved in various metabolic pathways, primarily through its interactions with enzymes such as hydroxylase and dioxygenase These enzymes facilitate the compound’s degradation and transformation, leading to changes in metabolic flux and metabolite levels
Transport and Distribution
The transport and distribution of 9,10-Anthracenediol within cells and tissues are influenced by its interactions with transporters and binding proteins. Studies have shown that the compound can be sequestered within xerogels, where it senses and reports from a distribution of microenvironments . These interactions are crucial for understanding the compound’s localization and accumulation within biological systems.
Subcellular Localization
9,10-Anthracenediol’s subcellular localization is influenced by its targeting signals and post-translational modifications. The compound can be directed to specific compartments or organelles within cells, where it exerts its effects on cellular function
Preparation Methods
Synthetic Routes and Reaction Conditions: anthracene-9,10-diol can be synthesized through the reduction of anthraquinone. The reduction process typically involves the use of hydrogen gas in the presence of a catalyst such as palladium on carbon (Pd/C) under controlled conditions . The reaction is carried out at elevated temperatures and pressures to ensure complete reduction.
Industrial Production Methods: In an industrial setting, the production of 9,10-anthracenediol follows similar principles but on a larger scale. The process involves the continuous flow of anthraquinone and hydrogen gas over a fixed bed of Pd/C catalyst. The reaction conditions are optimized to maximize yield and purity, with careful control of temperature, pressure, and flow rates .
Chemical Reactions Analysis
Types of Reactions: anthracene-9,10-diol undergoes various chemical reactions, including:
Oxidation: It can be oxidized back to anthraquinone using oxidizing agents such as potassium permanganate (KMnO4) or chromium trioxide (CrO3).
Reduction: Further reduction can lead to the formation of tetrahydroanthracene derivatives.
Substitution: The hydroxyl groups can participate in substitution reactions, forming ethers or esters when reacted with alkyl halides or acyl chlorides, respectively
Common Reagents and Conditions:
Oxidation: KMnO4 in an acidic medium.
Reduction: Hydrogen gas with Pd/C catalyst.
Substitution: Alkyl halides or acyl chlorides in the presence of a base such as pyridine
Major Products:
Oxidation: Anthraquinone.
Reduction: Tetrahydroanthracene derivatives.
Substitution: Ethers or esters of 9,10-anthracenediol
Scientific Research Applications
anthracene-9,10-diol has a wide range of applications in scientific research:
Comparison with Similar Compounds
9,10-Anthracenedione (Anthraquinone): A closely related compound that differs by having carbonyl groups instead of hydroxyl groups at the 9 and 10 positions.
9,10-Diphenylanthracene: Another derivative of anthracene, substituted with phenyl groups, known for its high fluorescence quantum yield.
Uniqueness: anthracene-9,10-diol is unique due to its dual hydroxyl groups, which impart distinct chemical reactivity and photophysical properties. This makes it particularly valuable in applications requiring specific interactions with light and in the synthesis of complex organic molecules .
Biological Activity
Anthracene-9,10-diol (C14H10O2) is a dihydroxy derivative of anthracene, notable for its unique biological properties and applications in various fields, including medicinal chemistry and photodynamic therapy. This article provides a comprehensive overview of the biological activity of this compound, supported by research findings, case studies, and data tables.
Chemical Structure and Properties
This compound is characterized by two hydroxyl groups at the 9 and 10 positions of the anthracene ring. This structural feature significantly influences its solubility and reactivity, particularly in alkaline solutions. The compound can be synthesized through several methods, including the reduction of 9,10-anthraquinone.
Antioxidant Properties
Research has indicated that this compound exhibits antioxidant activity , which is crucial for protecting biological systems from oxidative stress. Its ability to scavenge reactive oxygen species (ROS) suggests potential applications in preventing cellular damage associated with various diseases .
Photodynamic Therapy
This compound has been studied for its role in photodynamic therapy (PDT) . Upon light activation, it generates reactive oxygen species that can induce cytotoxic effects on cancer cells. This property makes it a candidate for cancer treatment strategies where localized destruction of malignant cells is desired .
DNA Interaction and Cytotoxicity
Studies have shown that this compound can interact with DNA, leading to cytotoxic effects. The compound's binding affinity to DNA was evaluated through thermal denaturation studies and unwinding assays. These investigations revealed that anthracene derivatives could occupy both major and minor grooves of DNA, potentially disrupting cellular functions and contributing to their cytotoxic properties .
Study on Anticancer Activity
A study explored a series of anthracene derivatives, including this compound, for their anticancer properties. The results demonstrated that compounds with structural modifications exhibited varying degrees of cytotoxicity against cancer cell lines. Notably, derivatives with longer side chains showed enhanced activity due to improved DNA binding characteristics .
Mutagenicity Assessment
Research assessing the mutagenic potential of anthracene derivatives found that this compound could induce mutations in certain bacterial strains. This raises considerations regarding its safety profile in therapeutic applications .
Data Tables
The following table summarizes key findings related to the biological activity of this compound compared to other related compounds.
Compound Name | Antioxidant Activity | Photodynamic Activity | DNA Binding Affinity | Cytotoxicity |
---|---|---|---|---|
This compound | Moderate | High | Strong | High |
Anthraquinone | Low | Moderate | Moderate | Moderate |
9-Hydroxyanthracene | Low | Low | Weak | Low |
1,8-Dihydroxyanthracene | Moderate | Low | Moderate | Moderate |
Properties
IUPAC Name |
anthracene-9,10-diol | |
---|---|---|
Source | PubChem | |
URL | https://pubchem.ncbi.nlm.nih.gov | |
Description | Data deposited in or computed by PubChem | |
InChI |
InChI=1S/C14H10O2/c15-13-9-5-1-2-6-10(9)14(16)12-8-4-3-7-11(12)13/h1-8,15-16H | |
Source | PubChem | |
URL | https://pubchem.ncbi.nlm.nih.gov | |
Description | Data deposited in or computed by PubChem | |
InChI Key |
PCFMUWBCZZUMRX-UHFFFAOYSA-N | |
Source | PubChem | |
URL | https://pubchem.ncbi.nlm.nih.gov | |
Description | Data deposited in or computed by PubChem | |
Canonical SMILES |
C1=CC=C2C(=C1)C(=C3C=CC=CC3=C2O)O | |
Source | PubChem | |
URL | https://pubchem.ncbi.nlm.nih.gov | |
Description | Data deposited in or computed by PubChem | |
Molecular Formula |
C14H10O2 | |
Source | PubChem | |
URL | https://pubchem.ncbi.nlm.nih.gov | |
Description | Data deposited in or computed by PubChem | |
DSSTOX Substance ID |
DTXSID4063664 | |
Record name | 9,10-Anthracenediol | |
Source | EPA DSSTox | |
URL | https://comptox.epa.gov/dashboard/DTXSID4063664 | |
Description | DSSTox provides a high quality public chemistry resource for supporting improved predictive toxicology. | |
Molecular Weight |
210.23 g/mol | |
Source | PubChem | |
URL | https://pubchem.ncbi.nlm.nih.gov | |
Description | Data deposited in or computed by PubChem | |
CAS No. |
4981-66-2 | |
Record name | Anthrahydroquinone | |
Source | CAS Common Chemistry | |
URL | https://commonchemistry.cas.org/detail?cas_rn=4981-66-2 | |
Description | CAS Common Chemistry is an open community resource for accessing chemical information. Nearly 500,000 chemical substances from CAS REGISTRY cover areas of community interest, including common and frequently regulated chemicals, and those relevant to high school and undergraduate chemistry classes. This chemical information, curated by our expert scientists, is provided in alignment with our mission as a division of the American Chemical Society. | |
Explanation | The data from CAS Common Chemistry is provided under a CC-BY-NC 4.0 license, unless otherwise stated. | |
Record name | 9,10-Dihydroxyanthracene | |
Source | ChemIDplus | |
URL | https://pubchem.ncbi.nlm.nih.gov/substance/?source=chemidplus&sourceid=0004981662 | |
Description | ChemIDplus is a free, web search system that provides access to the structure and nomenclature authority files used for the identification of chemical substances cited in National Library of Medicine (NLM) databases, including the TOXNET system. | |
Record name | 9,10-Anthracenediol | |
Source | EPA Chemicals under the TSCA | |
URL | https://www.epa.gov/chemicals-under-tsca | |
Description | EPA Chemicals under the Toxic Substances Control Act (TSCA) collection contains information on chemicals and their regulations under TSCA, including non-confidential content from the TSCA Chemical Substance Inventory and Chemical Data Reporting. | |
Record name | 9,10-Anthracenediol | |
Source | EPA DSSTox | |
URL | https://comptox.epa.gov/dashboard/DTXSID4063664 | |
Description | DSSTox provides a high quality public chemistry resource for supporting improved predictive toxicology. | |
Record name | 9,10-DIHYDROXYANTHRACENE | |
Source | FDA Global Substance Registration System (GSRS) | |
URL | https://gsrs.ncats.nih.gov/ginas/app/beta/substances/ETK5C5EZG6 | |
Description | The FDA Global Substance Registration System (GSRS) enables the efficient and accurate exchange of information on what substances are in regulated products. Instead of relying on names, which vary across regulatory domains, countries, and regions, the GSRS knowledge base makes it possible for substances to be defined by standardized, scientific descriptions. | |
Explanation | Unless otherwise noted, the contents of the FDA website (www.fda.gov), both text and graphics, are not copyrighted. They are in the public domain and may be republished, reprinted and otherwise used freely by anyone without the need to obtain permission from FDA. Credit to the U.S. Food and Drug Administration as the source is appreciated but not required. | |
Synthesis routes and methods I
Procedure details
Synthesis routes and methods II
Procedure details
Synthesis routes and methods III
Procedure details
Synthesis routes and methods IV
Procedure details
Synthesis routes and methods V
Procedure details
Retrosynthesis Analysis
AI-Powered Synthesis Planning: Our tool employs the Template_relevance Pistachio, Template_relevance Bkms_metabolic, Template_relevance Pistachio_ringbreaker, Template_relevance Reaxys, Template_relevance Reaxys_biocatalysis model, leveraging a vast database of chemical reactions to predict feasible synthetic routes.
One-Step Synthesis Focus: Specifically designed for one-step synthesis, it provides concise and direct routes for your target compounds, streamlining the synthesis process.
Accurate Predictions: Utilizing the extensive PISTACHIO, BKMS_METABOLIC, PISTACHIO_RINGBREAKER, REAXYS, REAXYS_BIOCATALYSIS database, our tool offers high-accuracy predictions, reflecting the latest in chemical research and data.
Strategy Settings
Precursor scoring | Relevance Heuristic |
---|---|
Min. plausibility | 0.01 |
Model | Template_relevance |
Template Set | Pistachio/Bkms_metabolic/Pistachio_ringbreaker/Reaxys/Reaxys_biocatalysis |
Top-N result to add to graph | 6 |
Feasible Synthetic Routes
Q1: What is the molecular formula and weight of 9,10-dihydroxyanthracene?
A1: The molecular formula of 9,10-dihydroxyanthracene is C14H10O2, and its molecular weight is 210.23 g/mol.
Q2: What spectroscopic data is available for 9,10-dihydroxyanthracene?
A2: [] The dichroic spectra of 9,10-dihydroxyanthracene have been measured in stretched polyvinyl alcohol sheets and compared with MO calculations to determine electronic band polarizations. [] This information can be useful for characterizing and quantifying the compound.
Q3: How does the structure of 9,10-dihydroxyanthracene relate to its properties?
A3: The presence of two hydroxyl groups at the 9,10 positions of the anthracene ring significantly influences the compound's reactivity and redox properties. [, ] These groups enable 9,10-dihydroxyanthracene to act as both a hydrogen donor and acceptor, contributing to its role in various photochemical and electrochemical reactions. [, ]
Q4: What is the role of 9,10-dihydroxyanthracene in the photoreduction of anthraquinones?
A4: 9,10-Dihydroxyanthracene is a common product in the photoreduction of anthraquinones. [, , , ] This process often involves hydrogen atom abstraction from solvents like ethanol by the excited state of anthraquinone derivatives, leading to the formation of semiquinone radicals and ultimately 9,10-dihydroxyanthracene. [, , ]
Q5: How do amines affect the photoreduction of anthraquinone to 9,10-dihydroxyanthracene?
A5: Amines, such as triethylamine (TEA), can act as electron donors in the photoreduction of anthraquinone. [, , ] The excited triplet state of anthraquinone can accept an electron from TEA, forming the anthraquinone radical anion and the TEA radical cation. [, ] This process ultimately leads to the formation of 9,10-dihydroxyanthracene. []
Q6: Can 9,10-dihydroxyanthracene be used in sensor applications?
A6: Yes, research indicates that 9,10-dihydroxyanthracene can be incorporated into sensor designs. [, ] For instance, it has shown promise as a sensitive layer on silicon nanowire field-effect transistors (SiNW-FETs) for detecting H2O2 and pH changes in physiological solutions. [] Additionally, it has been employed in a novel sensor platform called site selectively templated and tagged xerogels (SSTTX) for detecting analytes like 9-anthrol. []
Q7: How does 9,10-dihydroxyanthracene interact with lignin in pulping processes?
A7: 9,10-Dihydroxyanthracene and its oxidized form, anthraquinone, are known to participate in redox cycles during alkaline pulping processes, influencing delignification. [, , ] Specifically, anthraquinone can be reduced to 9,10-dihydroxyanthracene, which can then react with lignin model quinone methides. [] This interaction is believed to be crucial in anthraquinone-catalyzed delignification. []
Q8: Can 9,10-dihydroxyanthracene be used in battery applications?
A8: Research suggests potential for 9,10-dihydroxyanthracene and its derivatives in battery applications. [, ] For example, polymeric forms of anthraquinone, which can be electrochemically converted to 9,10-dihydroxyanthracene, have been investigated as electrode materials in aqueous lithium-ion batteries. [] These studies highlight the ability of the anthraquinone/9,10-dihydroxyanthracene redox couple to store charge. [, ]
Q9: What is the role of 9,10-dihydroxyanthracene in organic synthesis?
A9: 9,10-Dihydroxyanthracene can serve as a versatile intermediate in organic synthesis. [] Its structure allows for various transformations, including electrophilic aromatic substitution and oxidation reactions. [] Additionally, its derivatives, such as bis(perfluoroalkyl)-substituted anthracene diols, can be further modified via cross-coupling reactions to create extended π-conjugated systems. []
Q10: Is 9,10-dihydroxyanthracene stable in the presence of oxygen?
A10: 9,10-Dihydroxyanthracene is susceptible to oxidation in the presence of oxygen, reverting back to 9,10-anthraquinone. [] This sensitivity to oxygen is a crucial factor to consider when handling and storing the compound, particularly in applications where its fluorescence is desired. []
Q11: What factors can affect the stability of 9,10-dihydroxyanthracene in solution?
A11: The stability of 9,10-dihydroxyanthracene in solution can be affected by various factors, including solvent polarity, pH, temperature, and the presence of oxidizing agents. [, , , ] Understanding these factors is essential for optimizing reaction conditions and developing stable formulations.
Q12: Have there been any computational studies on 9,10-dihydroxyanthracene?
A12: Yes, computational chemistry methods, such as CNDO/S3 calculations, have been employed to study the electronic structure and reactivity of 9,10-dihydroxyanthracene and related systems. [] These calculations provide insights into the electron transfer processes and interactions with other molecules, contributing to a deeper understanding of its chemical behavior.
Q13: What is the environmental impact of 9,10-dihydroxyanthracene?
A13: While 9,10-dihydroxyanthracene itself has limited information available on its direct environmental impact, its use in industrial processes like pulping necessitates careful consideration. [, , ] Responsible waste management and exploration of alternative pulping methods are essential for minimizing potential negative environmental effects.
Disclaimer and Information on In-Vitro Research Products
Please be aware that all articles and product information presented on BenchChem are intended solely for informational purposes. The products available for purchase on BenchChem are specifically designed for in-vitro studies, which are conducted outside of living organisms. In-vitro studies, derived from the Latin term "in glass," involve experiments performed in controlled laboratory settings using cells or tissues. It is important to note that these products are not categorized as medicines or drugs, and they have not received approval from the FDA for the prevention, treatment, or cure of any medical condition, ailment, or disease. We must emphasize that any form of bodily introduction of these products into humans or animals is strictly prohibited by law. It is essential to adhere to these guidelines to ensure compliance with legal and ethical standards in research and experimentation.