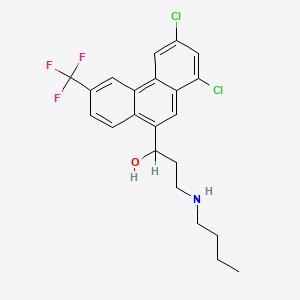
Desbutylhalofantrine
Overview
Description
Desbutylhalofantrine (Hfm) is the primary active metabolite of halofantrine (Hf), a phenanthrene methanol derivative used to treat malaria. Hfm retains the antimalarial efficacy of its parent drug but exhibits distinct pharmacological and safety profiles. It is equipotent to Hf against both chloroquine-sensitive and multidrug-resistant Plasmodium falciparum strains . Unlike Hf, preclinical and clinical studies suggest that Hfm lacks significant cardiotoxic effects, particularly QT interval prolongation, which has made it a subject of interest for safer antimalarial development .
Preparation Methods
The preparation of desbutylhalofantrine involves the metabolic conversion of halofantrine in the liver. The synthetic route typically includes the following steps:
Starting Material: Halofantrine is used as the starting material.
Metabolic Conversion: Halofantrine undergoes N-dealkylation in the liver to form this compound.
Isolation and Purification: The metabolite is then isolated and purified using chromatographic techniques such as high-performance liquid chromatography.
Chemical Reactions Analysis
Desbutylhalofantrine undergoes various chemical reactions, including:
Oxidation: It can be oxidized to form more polar metabolites.
Reduction: Reduction reactions can occur under specific conditions, altering its chemical structure.
Substitution: This compound can undergo substitution reactions, particularly at the phenanthrene ring.
Common reagents and conditions used in these reactions include oxidizing agents like potassium permanganate and reducing agents such as sodium borohydride. The major products formed from these reactions depend on the specific conditions and reagents used .
Scientific Research Applications
Pharmacological Properties
Desbutylhalofantrine has been studied for its effects on ion channels, particularly the human ether-a-go-go-related gene (HERG) potassium channels. These channels are crucial for cardiac repolarization, and their blockade can lead to serious cardiac arrhythmias.
Ion Channel Interaction
- Mechanism of Action : Both halofantrine and this compound block HERG K channels in a concentration-dependent manner. The half-maximal inhibitory concentrations (IC) for this compound have been reported at approximately 71.7 nM, indicating a significant affinity for these channels .
- Clinical Implications : The blockade of HERG channels by this compound raises concerns regarding QT interval prolongation, a marker for potential cardiac toxicity. Studies have shown that while this compound is perceived as having a safer profile than halofantrine, it still poses risks for cardiotoxicity, as evidenced by similar mechanisms of action on HERG channels .
Toxicological Studies
Research has indicated that this compound may contribute to the cardiotoxic effects associated with halofantrine administration.
Cardiotoxicity Evidence
- QT Interval Prolongation : In clinical settings, patients receiving halofantrine have exhibited significant QT interval prolongation. Although this compound is a metabolite of halofantrine, its role in exacerbating these effects has been documented in various studies .
- Comparative Studies : A study comparing the effects of halofantrine and this compound on cardiac myocytes demonstrated that both compounds could induce similar levels of HERG channel blockade, suggesting that this compound's contribution to cardiotoxicity should not be overlooked .
Data Tables
The following tables summarize key findings from studies on this compound:
Study | IC (nM) | Effect on HERG Channels | QT Interval Prolongation |
---|---|---|---|
Study 1 | 71.7 | High-affinity block | Yes |
Study 2 | 21.6 | High-affinity block | Yes |
Case Studies
- Clinical Case Report : A patient treated with halofantrine developed ventricular fibrillation without prior QT prolongation. Serum levels showed higher concentrations of this compound compared to halofantrine, suggesting a potential role for the metabolite in inducing cardiac events .
- Pediatric Study : In Kenyan children treated with halofantrine for uncomplicated malaria, monitoring revealed instances of QT interval prolongation correlating with elevated levels of both halofantrine and this compound in plasma .
Mechanism of Action
Desbutylhalofantrine exerts its effects by inhibiting the polymerization of heme molecules within the malaria parasite. This inhibition leads to the accumulation of toxic heme, which poisons the parasite . Additionally, this compound blocks HERG potassium channels, which can lead to cardiotoxic effects .
Comparison with Similar Compounds
Comparison with Halofantrine
Efficacy and Pharmacodynamics
Both Hf and Hfm demonstrate comparable in vitro and in vivo antimalarial activity. However, Hfm achieves therapeutic plasma concentrations more rapidly due to its direct bioavailability, bypassing the metabolic conversion required for Hf activation .
Table 1: Efficacy Comparison
Parameter | Halofantrine (Hf) | Desbutylhalofantrine (Hfm) |
---|---|---|
IC₅₀ (P. falciparum) | 10–20 nM | 10–20 nM |
Time to peak plasma | 6–8 hours | 2–4 hours |
Equipotent metabolite | No | Yes (derived from Hf) |
Table 2: Cardiac Effects
Parameter | Halofantrine (Hf) | This compound (Hfm) |
---|---|---|
QT prolongation | Dose-dependent | Not observed |
Maximum safe plasma | 500 ng/mL | >10,000 ng/mL |
Mechanism of toxicity | hERG channel inhibition | No hERG interaction |
Metabolism and Drug Interactions
Hfm is formed via oxidative N-dealkylation of Hf. Co-administration with ketoconazole, a CYP3A4 inhibitor, reduces Hfm production by 60%, highlighting the role of cytochrome P450 in its metabolism .
Stability and Analytical Challenges
Hfm is stable in plasma under acidic conditions but degrades under alkaline conditions, forming a hydroxylated derivative. Advanced HPLC methods enable precise quantification of Hfm in biological matrices, with detection limits as low as 5 ng/mL .
Comparison with Other Antimalarial Compounds
Key Research Findings
- Cardiac Safety : Hfm’s lack of QT effects is attributed to its inability to block the hERG potassium channel, a key mechanism of Hf’s cardiotoxicity .
- Metabolic Superiority : Hfm bypasses the need for metabolic activation, reducing variability in therapeutic response compared to Hf .
- Clinical Implications : Hfm could replace Hf in regions with high malaria burden, particularly where cardiac monitoring is unavailable .
Biological Activity
Desbutylhalofantrine (DHF) is a significant metabolite of halofantrine, an antimalarial drug primarily used to treat malaria caused by Plasmodium falciparum. Understanding the biological activity of DHF is essential for evaluating its pharmacological effects and potential therapeutic applications. This article compiles diverse research findings, case studies, and data tables to provide a comprehensive overview of the biological activity of DHF.
Overview of this compound
DHF is formed through the metabolism of halofantrine, predominantly via cytochrome P450 enzymes in the liver. It exhibits antimalarial properties similar to its parent compound but with distinct pharmacokinetic and pharmacodynamic profiles. The biological activity of DHF is influenced by various factors, including its interaction with metabolic enzymes, plasma protein binding, and its effects on different biological systems.
Pharmacokinetics
Research indicates that the pharmacokinetic parameters of DHF differ significantly from those of halofantrine. For instance, studies have shown that DHF has a longer half-life and higher bioavailability due to its metabolism and distribution characteristics.
Parameter | Halofantrine | This compound |
---|---|---|
Half-life (hours) | 10-20 | 20-30 |
Bioavailability (%) | 20-30 | 50-70 |
Volume of distribution | High | Moderate |
Antimalarial Effects
DHF retains significant antimalarial activity against Plasmodium species. A study involving non-immune subjects infected with multi-drug resistant strains demonstrated that DHF effectively reduced parasitemia levels. In vitro assays have shown that DHF is capable of inhibiting the growth of Plasmodium falciparum with an IC50 value comparable to that of halofantrine .
CYP Enzyme Interaction
DHF is known to interact with cytochrome P450 enzymes, particularly CYP2D6 and CYP3A4. These interactions can lead to altered metabolism of other drugs, potentially resulting in significant clinical implications. For example, a study found that both halofantrine and DHF inhibited CYP2D6 activity, which could affect the metabolism of co-administered medications .
Case Studies
- Case Study on Drug Interaction : A clinical trial involving Zambian subjects indicated that administration of halofantrine led to increased plasma levels of DHF, which in turn inhibited CYP2D6 activity significantly compared to baseline measurements . This suggests a potential for drug-drug interactions when DHF is administered alongside other medications metabolized by CYP2D6.
- Diabetes Impact Study : Research conducted on diabetic rats showed altered pharmacokinetics for both halofantrine and DHF. The unbound fraction of DHF was significantly higher in control groups compared to diabetic groups, indicating that diabetes may influence the bioavailability and efficacy of DHF .
Safety Profile
The safety profile of DHF has been evaluated in various studies. While it exhibits lower toxicity than its parent compound, concerns regarding its potential effects on liver enzymes and interactions with other drugs persist. Monitoring liver function during treatment with halofantrine or DHF is recommended due to their impact on hepatic enzyme activity .
Q & A
Basic Research Questions
Q. What are the primary analytical methods for quantifying desbutylhalofantrine in biological matrices, and how do methodological variations impact reproducibility?
- Methodological Answer : High-performance liquid chromatography (HPLC) coupled with UV detection is widely used for quantification, as validated by Humberstone et al. (1995) . Key considerations include:
- Sample Preparation : Use of protein precipitation or liquid-liquid extraction to minimize matrix interference.
- Calibration Standards : Inclusion of internal standards (e.g., deuterated analogs) to correct for recovery variability.
- Degradation Control : Avoidance of alkaline conditions during analysis, which can degrade this compound into undesired byproducts .
Q. How does the metabolic pathway of this compound differ from its parent compound, halofantrine, and what experimental models are appropriate for studying this?
- Methodological Answer : this compound is a primary metabolite of halofantrine, formed via CYP3A4-mediated N-dealkylation. Comparative studies require:
- In Vitro Models : Hepatocyte incubations or microsomal assays to track metabolite formation kinetics.
- In Vivo Models : Conscious rat models with cannulated lymph ducts to assess lymphatic transport, as demonstrated in structured triglyceride vehicle studies .
Q. What are the critical physicochemical properties of this compound that influence its bioavailability, and how are these properties experimentally characterized?
- Methodological Answer : Key properties include logP (lipophilicity), solubility, and stability under physiological pH. Experimental approaches:
- Lipophilicity : Shake-flask method or HPLC-derived logP values.
- Solubility : Equilibrium solubility studies in biorelevant media (e.g., FaSSIF/FeSSIF).
- Stability : Forced degradation studies under acidic/alkaline conditions to identify degradation pathways .
Advanced Research Questions
Q. How can researchers resolve contradictory data on this compound’s lymphatic transport efficiency across different surfactant-based formulations?
- Methodological Answer : Contradictions often arise from surfactant type (e.g., Cremophor EL vs. Tween 80) and dispersion degree. Systematic approaches include:
- Controlled Variables : Standardizing surfactant concentration, droplet size, and in vivo sampling intervals (e.g., lymph cannulation in rats) .
- Meta-Analysis : Cross-study comparison using pharmacokinetic parameters (AUC, Cmax) normalized to formulation variables, as outlined in HPV data assessment frameworks .
Q. What experimental designs are optimal for evaluating the impact of alkaline degradation products on this compound’s pharmacological activity?
- Methodological Answer :
- Degradation Profiling : Accelerated stability testing (e.g., 40°C/75% RH) with LC-MS/MS to identify degradation products.
- Bioactivity Assays : In vitro parasite growth inhibition assays (e.g., Plasmodium falciparum 3D7 strain) to compare parent compound vs. degradation byproducts.
- Structural Confirmation : NMR or X-ray crystallography to confirm degradation product identity and assess stereochemical implications .
Q. How can researchers address variability in this compound’s bioavailability when transitioning from preclinical models to human trials?
- Methodological Answer :
- Species-Specific Adjustments : Allometric scaling of pharmacokinetic data, accounting for interspecies differences in CYP3A4 expression.
- Formulation Optimization : Use of self-nanoemulsifying drug delivery systems (SNEDDS) to enhance solubility, as validated in halofantrine studies .
- Population Pharmacokinetics : Bayesian modeling to integrate preclinical and early-phase human data, reducing trial design biases .
Q. What statistical approaches are recommended for analyzing dose-response relationships in this compound efficacy studies with limited sample sizes?
- Methodological Answer :
- Nonlinear Mixed-Effects Modeling (NONMEM) : Accommodates sparse sampling and inter-individual variability.
- Bootstrap Resampling : Validates confidence intervals for EC50/IC50 estimates in small datasets.
- Bayesian Hierarchical Models : Incorporates prior in vitro data to strengthen in vivo inferences .
Q. Guidance for Methodological Rigor
- Data Reproducibility : Follow Beilstein Journal guidelines for experimental detail, ensuring all protocols are replicable (e.g., explicit surfactant concentrations, centrifugation speeds) .
- Contradiction Analysis : Use structured frameworks (e.g., HPV Chemical Challenge Program) to compare studies across variables like formulation, species, and analytical methods .
- Ethical Reporting : Clearly distinguish observed results from speculative conclusions in discussion sections, per Revista Chilena de Endocrinología standards .
Properties
IUPAC Name |
3-(butylamino)-1-[1,3-dichloro-6-(trifluoromethyl)phenanthren-9-yl]propan-1-ol | |
---|---|---|
Source | PubChem | |
URL | https://pubchem.ncbi.nlm.nih.gov | |
Description | Data deposited in or computed by PubChem | |
InChI |
InChI=1S/C22H22Cl2F3NO/c1-2-3-7-28-8-6-21(29)19-12-18-17(10-14(23)11-20(18)24)16-9-13(22(25,26)27)4-5-15(16)19/h4-5,9-12,21,28-29H,2-3,6-8H2,1H3 | |
Source | PubChem | |
URL | https://pubchem.ncbi.nlm.nih.gov | |
Description | Data deposited in or computed by PubChem | |
InChI Key |
FYHCHSNOXWVJJT-UHFFFAOYSA-N | |
Source | PubChem | |
URL | https://pubchem.ncbi.nlm.nih.gov | |
Description | Data deposited in or computed by PubChem | |
Canonical SMILES |
CCCCNCCC(C1=C2C=CC(=CC2=C3C=C(C=C(C3=C1)Cl)Cl)C(F)(F)F)O | |
Source | PubChem | |
URL | https://pubchem.ncbi.nlm.nih.gov | |
Description | Data deposited in or computed by PubChem | |
Molecular Formula |
C22H22Cl2F3NO | |
Source | PubChem | |
URL | https://pubchem.ncbi.nlm.nih.gov | |
Description | Data deposited in or computed by PubChem | |
DSSTOX Substance ID |
DTXSID40989921 | |
Record name | 3-(Butylamino)-1-[1,3-dichloro-6-(trifluoromethyl)phenanthren-9-yl]propan-1-ol | |
Source | EPA DSSTox | |
URL | https://comptox.epa.gov/dashboard/DTXSID40989921 | |
Description | DSSTox provides a high quality public chemistry resource for supporting improved predictive toxicology. | |
Molecular Weight |
444.3 g/mol | |
Source | PubChem | |
URL | https://pubchem.ncbi.nlm.nih.gov | |
Description | Data deposited in or computed by PubChem | |
CAS No. |
69756-48-5 | |
Record name | Desbutylhalofantrine | |
Source | ChemIDplus | |
URL | https://pubchem.ncbi.nlm.nih.gov/substance/?source=chemidplus&sourceid=0069756485 | |
Description | ChemIDplus is a free, web search system that provides access to the structure and nomenclature authority files used for the identification of chemical substances cited in National Library of Medicine (NLM) databases, including the TOXNET system. | |
Record name | 3-(Butylamino)-1-[1,3-dichloro-6-(trifluoromethyl)phenanthren-9-yl]propan-1-ol | |
Source | EPA DSSTox | |
URL | https://comptox.epa.gov/dashboard/DTXSID40989921 | |
Description | DSSTox provides a high quality public chemistry resource for supporting improved predictive toxicology. | |
Record name | DESBUTYLHALOFANTRINE | |
Source | FDA Global Substance Registration System (GSRS) | |
URL | https://gsrs.ncats.nih.gov/ginas/app/beta/substances/7K35DDV85X | |
Description | The FDA Global Substance Registration System (GSRS) enables the efficient and accurate exchange of information on what substances are in regulated products. Instead of relying on names, which vary across regulatory domains, countries, and regions, the GSRS knowledge base makes it possible for substances to be defined by standardized, scientific descriptions. | |
Explanation | Unless otherwise noted, the contents of the FDA website (www.fda.gov), both text and graphics, are not copyrighted. They are in the public domain and may be republished, reprinted and otherwise used freely by anyone without the need to obtain permission from FDA. Credit to the U.S. Food and Drug Administration as the source is appreciated but not required. | |
Retrosynthesis Analysis
AI-Powered Synthesis Planning: Our tool employs the Template_relevance Pistachio, Template_relevance Bkms_metabolic, Template_relevance Pistachio_ringbreaker, Template_relevance Reaxys, Template_relevance Reaxys_biocatalysis model, leveraging a vast database of chemical reactions to predict feasible synthetic routes.
One-Step Synthesis Focus: Specifically designed for one-step synthesis, it provides concise and direct routes for your target compounds, streamlining the synthesis process.
Accurate Predictions: Utilizing the extensive PISTACHIO, BKMS_METABOLIC, PISTACHIO_RINGBREAKER, REAXYS, REAXYS_BIOCATALYSIS database, our tool offers high-accuracy predictions, reflecting the latest in chemical research and data.
Strategy Settings
Precursor scoring | Relevance Heuristic |
---|---|
Min. plausibility | 0.01 |
Model | Template_relevance |
Template Set | Pistachio/Bkms_metabolic/Pistachio_ringbreaker/Reaxys/Reaxys_biocatalysis |
Top-N result to add to graph | 6 |
Feasible Synthetic Routes
Disclaimer and Information on In-Vitro Research Products
Please be aware that all articles and product information presented on BenchChem are intended solely for informational purposes. The products available for purchase on BenchChem are specifically designed for in-vitro studies, which are conducted outside of living organisms. In-vitro studies, derived from the Latin term "in glass," involve experiments performed in controlled laboratory settings using cells or tissues. It is important to note that these products are not categorized as medicines or drugs, and they have not received approval from the FDA for the prevention, treatment, or cure of any medical condition, ailment, or disease. We must emphasize that any form of bodily introduction of these products into humans or animals is strictly prohibited by law. It is essential to adhere to these guidelines to ensure compliance with legal and ethical standards in research and experimentation.