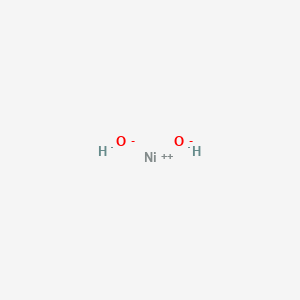
Nickel hydroxide
Overview
Description
Nickel (II) hydroxide, represented by the chemical formula Ni(OH)2, is an inorganic compound that displays intriguing properties and has wide-ranging industrial applications . It is a lime-green solid that dissolves with decomposition in ammonia and amines and is attacked by acids . It is electroactive, being converted to the Ni(III) oxy-hydroxide, leading to widespread applications in rechargeable batteries .
Synthesis Analysis
Nickel (II) hydroxide is frequently synthesized via the reaction of a nickel (II) salt such as nickel (II) sulfate with a strong base like sodium hydroxide . This reaction gives rise to Nickel (II) hydroxide precipitate and a sodium salt . In addition, nickel hydroxide nanosheets with a hexagonal island shape are synthesized via a surfactant-assisted method .Molecular Structure Analysis
Nickel (II) hydroxide has two well-characterized polymorphs, α and β . The α structure consists of Ni(OH)2 layers with intercalated anions or water . The β form adopts a hexagonal close-packed structure of Ni2+ and OH- ions . In the presence of water, the α polymorph typically recrystallizes to the β form .Chemical Reactions Analysis
Nickel (II) hydroxide is used in various chemical reactions. For instance, it is used in the production of rechargeable batteries, particularly nickel-metal hydride (Ni-MH) batteries . It serves as the active material in the positive electrode in these batteries .Physical and Chemical Properties Analysis
Nickel (II) hydroxide is typically seen as a green crystalline solid at room temperature . It’s largely insoluble in water but dissolves in acids to produce corresponding nickel salts . As a hydroxide, it exhibits basic properties, reacting with acids in typical acid-base reactions .Scientific Research Applications
Nanostructural Control for Enhanced Electrochemical Activity : High-surface-area β-Ni(OH)₂ demonstrates improved electrochemical properties, especially in rechargeable Ni-based alkaline batteries. Nanostructured nickel hydroxide exhibits better charge capacity and superior electrochemical activity (Sakai, Miyazaki, & Kijima, 2008).
Versatile Applications : this compound is crucial in various engineering applications, notably in batteries. Its structures, synthesis methods, and physical properties have been extensively reviewed, highlighting its significance in multiple technological fields (Hall, Lockwood, Bock, & Macdougall, 2015).
Supercapacitor Applications : A multidimensional rational design involving nickel-iron sulfide and carbon nanotubes on diatomite has been studied for supercapacitors. This approach improves electrochemical performance by providing uniform dispersion and increasing active sites (Li et al., 2021).
Electrochemical Pseudocapacitors : Amorphous this compound nanospheres show promise as high-performance electrode materials for electrochemical capacitors. They exhibit high capacitance, energy density, and long cycle life (Li et al., 2013).
Enhanced Electrochemical Activity in Alkaline Batteries : 3D flowerlike α-Nickel Hydroxide, with its superior electrochemical properties compared to the β-form, shows potential in rechargeable Ni-based alkaline batteries (Xu et al., 2008).
Dynamic Processes in Energy Storage Devices : Understanding the dynamic processes at the solid-liquid interface of nickel (oxy)hydroxide is crucial for enhancing reaction kinetics in supercapacitors and oxygen-evolution catalysts (Deng et al., 2017).
Alkaline Secondary Cells and Hybrid Supercapacitors : β-Ni(OH)₂ is used as an active material in these applications, and its effectiveness and characteristics have been extensively studied, revealing insights into its electrochemical performance (Kovalenko & Kotok, 2017).
Applications in Urea Electrolysis : Two-dimensional this compound nanosheets have shown potential in applications such as urea-rich wastewater remediation, hydrogen production, and fuel cells due to their ability to promote the urea electrolysis reaction (Wang, Yan, & Botte, 2011).
Electrocatalysis and Electroanalysis : Nickel hydroxides are used in electrocatalysis towards small molecules, serving a critical role in areas like fuel cells, energy storage, and electrochemical sensors (Miao et al., 2014).
Supercapacitor Performance : this compound nanosheets synthesized by a simple microwave-assisted heating method show great potential as electrode materials for supercapacitors due to their high specific capacitance and cycling stability (Mondal et al., 2014).
Mechanism of Action
Safety and Hazards
Properties
CAS No. |
11113-74-9 |
---|---|
Molecular Formula |
HNiO- |
Molecular Weight |
75.701 g/mol |
IUPAC Name |
nickel;hydroxide |
InChI |
InChI=1S/Ni.H2O/h;1H2/p-1 |
InChI Key |
SPIFDSWFDKNERT-UHFFFAOYSA-M |
SMILES |
[OH-].[OH-].[Ni+2] |
Canonical SMILES |
[OH-].[Ni] |
Color/Form |
Black powder Green crystals, hexagonal Light green microcrystalline powde |
density |
4.1 at 68 °F (USCG, 1999) 4.1 |
flash_point |
Ignites in air at about 752.0° F (USCG, 1999) |
melting_point |
446 °F (USCG, 1999) Decomposes at 230 °C |
11113-74-9 12054-48-7 |
|
physical_description |
Nickel hydroxide appears as a fine green powder. Slightly soluble in water and denser than water. Primary hazard is threat to the environment. Immediate steps should be taken to limit spread to the environment. |
Pictograms |
Irritant; Health Hazard; Environmental Hazard |
solubility |
SOL IN DIL ACIDS, IN AMMONIA SOL IN AMMONIUM HYDROXIDE, ACIDS 0.013 G/100 CC COLD WATER |
Synonyms |
nickel hydroxide (II) nickel hydroxide (III) nickel(II)hydroxide NiOH2 |
Origin of Product |
United States |
Synthesis routes and methods
Procedure details
Retrosynthesis Analysis
AI-Powered Synthesis Planning: Our tool employs the Template_relevance Pistachio, Template_relevance Bkms_metabolic, Template_relevance Pistachio_ringbreaker, Template_relevance Reaxys, Template_relevance Reaxys_biocatalysis model, leveraging a vast database of chemical reactions to predict feasible synthetic routes.
One-Step Synthesis Focus: Specifically designed for one-step synthesis, it provides concise and direct routes for your target compounds, streamlining the synthesis process.
Accurate Predictions: Utilizing the extensive PISTACHIO, BKMS_METABOLIC, PISTACHIO_RINGBREAKER, REAXYS, REAXYS_BIOCATALYSIS database, our tool offers high-accuracy predictions, reflecting the latest in chemical research and data.
Strategy Settings
Precursor scoring | Relevance Heuristic |
---|---|
Min. plausibility | 0.01 |
Model | Template_relevance |
Template Set | Pistachio/Bkms_metabolic/Pistachio_ringbreaker/Reaxys/Reaxys_biocatalysis |
Top-N result to add to graph | 6 |
Feasible Synthetic Routes
Disclaimer and Information on In-Vitro Research Products
Please be aware that all articles and product information presented on BenchChem are intended solely for informational purposes. The products available for purchase on BenchChem are specifically designed for in-vitro studies, which are conducted outside of living organisms. In-vitro studies, derived from the Latin term "in glass," involve experiments performed in controlled laboratory settings using cells or tissues. It is important to note that these products are not categorized as medicines or drugs, and they have not received approval from the FDA for the prevention, treatment, or cure of any medical condition, ailment, or disease. We must emphasize that any form of bodily introduction of these products into humans or animals is strictly prohibited by law. It is essential to adhere to these guidelines to ensure compliance with legal and ethical standards in research and experimentation.