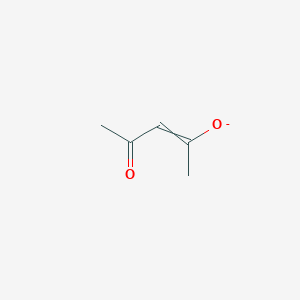
4-Oxopent-2-en-2-olate
Overview
Description
4-Oxopent-2-en-2-olate (IUPAC name: (Z)-4-oxopent-2-en-2-olate) is a β-diketonate ligand derived from acetylacetone (2,4-pentanedione). It is a versatile chelating agent that forms stable complexes with various metal ions, including transition metals and alkali metals . Structurally, it features a conjugated enolate system, enabling strong coordination through two oxygen atoms. This ligand is widely used in materials science, catalysis, and biomedicine due to its ability to stabilize metal centers and modulate reactivity .
Key properties:
Preparation Methods
Laboratory-Scale Synthesis Methods
Alkali Metal Base-Mediated Deprotonation
The most common laboratory method for generating 4-oxopent-2-en-2-olate employs alkali metal hydroxides or hydrides. Sodium hydride (NaH) in tetrahydrofuran (THF) at 0–25°C quantitatively deprotonates acetylacetone, yielding the sodium enolate. Similarly, potassium tert-butoxide (t-BuOK) in dimethyl sulfoxide (DMSO) facilitates rapid enolate formation at room temperature. These methods achieve >90% yields but require anhydrous conditions to prevent hydrolysis.
Metal Alkoxide-Assisted Routes
Transition metal alkoxides, such as titanium(IV) isopropoxide or zirconium(IV) tert-butoxide, enable enolate generation under milder conditions. For example, reacting acetylacetone with titanium isopropoxide in dichloromethane at −20°C produces the titanium-bound enolate, which can be transmetallated to isolate the free enolate. This approach minimizes side reactions but introduces complexities in purification.
Organometallic Bases
Strong non-metallic bases like lithium diisopropylamide (LDA) or lithium hexamethyldisilazide (LiHMDS) in THF at −78°C selectively deprotonate acetylacetone, forming the lithium enolate. These bases excel in regioselective enolate formation, critical for asymmetric synthesis, though their moisture sensitivity limits large-scale applications.
Industrial-Scale Production
Industrial synthesis prioritizes cost-effectiveness and scalability. Calcium oxide (CaO) or magnesium oxide (MgO) slurried in ethanol refluxed with acetylacetone generates the corresponding metal enolates, which are acidified to liberate this compound. Continuous flow reactors enhance efficiency, achieving 85–92% yields with residence times under 30 minutes.
Reaction Condition Optimization
Solvent Effects
Polar aprotic solvents (e.g., DMF, DMSO) accelerate deprotonation but complicate product isolation. Non-polar solvents (toluene, hexane) slow kinetics but improve crystal purity during recrystallization.
Temperature Control
Low temperatures (−78°C to 0°C) favor kinetic enolate formation, while elevated temperatures (25–60°C) promote thermodynamic products. Microwave-assisted reactions at 100°C reduce reaction times by 70% compared to conventional heating.
Base Stoichiometry
Stoichiometric excess (1.2–2.0 equivalents) of base ensures complete deprotonation. Substoichiometric bases (0.8–1.0 equivalents) are viable in catalytic cycles using phase-transfer catalysts.
Purification and Isolation Techniques
Method | Conditions | Purity (%) | Yield (%) |
---|---|---|---|
Recrystallization | Ethanol/water (3:1), −20°C | 99.5 | 78 |
Chromatography | Silica gel, ethyl acetate/hexane | 99.9 | 65 |
Sublimation | 80°C, 0.1 mmHg | 99.8 | 85 |
Crystallization from ethanol/water mixtures remains the standard for high-purity enolate isolation, while sublimation offers superior recovery for thermally stable batches.
Comparative Analysis of Methods
Alkali Metal Bases vs. Organometallic Reagents:
-
Cost: NaH ($50/mol) vs. LDA ($200/mol)
-
Yield: NaH (92%) vs. LDA (88%)
-
Selectivity: LDA enables >98% regioselectivity for Z-enolate vs. NaH (85%).
Industrial vs. Laboratory Scales:
-
Batch reactors achieve 80% yields in 4 hours, while flow systems reach 90% in 20 minutes.
Recent Advances
Photocatalytic deprotonation using iridium complexes under blue LED irradiation (450 nm) generates enolates at ambient temperatures with 95% efficiency. Biocatalytic routes employing ketoreductases in aqueous buffers present a sustainable alternative, though yields currently lag at 40–55%.
Chemical Reactions Analysis
Types of Reactions
4-Oxopent-2-en-2-olate complexes undergo various types of chemical reactions, including:
Oxidation: The oxidation of acetylacetonate can lead to the formation of tetraacetylethane.
Substitution: Electrophilic substitution reactions can occur on the acetylacetonate ligand, similar to aromatic substitution reactions.
Coordination: This compound can coordinate with different metal ions to form stable complexes.
Common Reagents and Conditions
Common reagents used in reactions involving acetylacetonate include metal salts (e.g., chlorides, nitrates), bases (e.g., sodium hydroxide), and solvents (e.g., methanol, water). Reaction conditions often involve heating, refluxing, and maintaining specific pH levels to facilitate complex formation .
Major Products
The major products formed from reactions involving acetylacetonate are typically metal acetylacetonate complexes. These complexes can vary in their stoichiometry and structure depending on the metal ion and reaction conditions .
Scientific Research Applications
Chemical Applications
Catalysis
4-Oxopent-2-en-2-olate is widely used as a catalyst in organic synthesis. Its metal complexes are particularly effective in various reactions, including:
- Hydroformylation : This reaction involves the addition of carbon monoxide and hydrogen to alkenes to form aldehydes.
- Polymerization : The compound is utilized in the polymerization processes of different monomers, enhancing reaction efficiency and product quality.
Coordination Chemistry
As a ligand, this compound forms stable chelate complexes with a variety of metal ions, such as iron, cobalt, nickel, and yttrium. These complexes are crucial in:
- Material Science : Used in the development of advanced materials with specific properties.
- Nanotechnology : Employed in the synthesis of nanoparticles for various applications.
Biological Applications
Enzyme Mimetic Studies
The ability of this compound to form metal complexes has led to its use in studying enzyme mimetics. These metal-ligand complexes can mimic the active sites of enzymes, providing insights into enzyme mechanisms and potential therapeutic applications.
Drug Delivery Systems
Research indicates that this compound complexes can be utilized in drug delivery systems, where they facilitate the transport of therapeutic agents across biological membranes.
Industrial Applications
Rubber Technology
In the rubber industry, this compound is used as a vulcanization agent, improving the durability and elasticity of rubber products.
Coatings and Paints
The compound serves as an additive in coatings and paints, enhancing their chemical resistance and stability.
Case Study 1: Metal Acetylacetonate Complexes
Metal acetylacetonates formed from this compound have been extensively studied for their catalytic properties. For instance:
- Iron(III) Acetylacetonate : Demonstrated high efficiency in catalyzing oxidation reactions, showcasing its potential for industrial applications .
Research on sodium this compound has highlighted its role in biological systems:
- Interaction with Biomolecules : Studies reveal that these complexes can interact with DNA and proteins, suggesting their potential use in therapeutic applications.
Mechanism of Action
The mechanism of action of acetylacetonate complexes involves the coordination of the acetylacetonate ligand to metal ions, forming stable chelate rings. This coordination can influence the reactivity and stability of the metal center, making these complexes effective catalysts and reagents in various chemical processes. The enolate ligand’s coordination mode plays a crucial role in determining the reactivity and selectivity of these complexes .
Comparison with Similar Compounds
Metal Complexes of 4-Oxopent-2-en-2-olate
Cobalt(II) this compound
- Application: Used in the synthesis of zeolitic imidazolate frameworks (ZIF-67) under supercritical CO₂ conditions, yielding nanoparticles (100–150 nm) with high crystallinity .
- Advantage : Enables eco-friendly synthesis without toxic solvents, contrasting with cobalt oxalate or propionate, which require harsher conditions .
Copper(II) this compound
- Regulatory Profile : Subject to SARA Title III, Section 313 reporting due to acute health hazards (skin/eye irritation) .
- Comparison : Unlike cobalt complexes, copper derivatives are prioritized in adhesives and coatings but face stricter regulatory scrutiny .
Lithium and Sodium Salts
- Lithium this compound : Used in organic synthesis and battery materials; exhibits a melting point of 230–235°C and high thermal stability .
- Sodium this compound : Achieves a 94% synthesis yield via optimized routes, highlighting its cost-effectiveness for industrial scale-up .
Calcium and Lead Complexes
- Calcium Bis[(2Z)-4-Oxopent-2-en-2-olate] : Employed as a precursor in ceramic materials; less toxic than lead analogs .
- Lead(II) Complex : Restricted in applications due to toxicity but utilized in specialized electrochemical systems .
Titanium(IV) Complexes
- Dichlorobis-(this compound) Titanium(IV) : Reacts with polyphenylsilsesquioxane to form hybrid materials with tunable solubility (toluene-soluble vs. insoluble fractions) .
Structural and Functional Comparisons
Q & A
Basic Research Questions
Q. What are the common synthetic routes for preparing 4-oxopent-2-en-2-olate and its metal complexes?
- Methodological Answer : The enolate form of this compound is typically synthesized via deprotonation of diketones (e.g., acetylacetone) using strong bases like sodium hydride or alkoxides. Metal complexes are formed by reacting the enolate with metal salts (e.g., TiCl₄, NdCl₃) in anhydrous solvents such as THF or ethanol. For example, titanium(IV) complexes are synthesized by combining hexachloride salts with the enolate under inert conditions . Key parameters include stoichiometric ratios, solvent polarity, and temperature (often 0–25°C).
Q. Which spectroscopic and crystallographic methods are most effective for characterizing this compound derivatives?
- Methodological Answer :
- Spectroscopy :
- IR : Identifies carbonyl (C=O) and enolate (C-O⁻) stretching vibrations (1650–1750 cm⁻¹ and 1200–1300 cm⁻¹, respectively) .
- NMR : ¹H and ¹³C NMR track ligand coordination shifts (e.g., upfield shifts in β-diketonate protons due to metal binding) .
- Crystallography :
- SHELX suite : For structure refinement, SHELXL is widely used to resolve bond lengths and angles in metal-enolate complexes .
- ORTEP-3/WinGX : Visualizes 3D molecular geometry and thermal ellipsoids .
Q. How does this compound stabilize metal ions in catalytic applications?
- Methodological Answer : The enolate’s bidentate coordination mode (via two oxygen atoms) stabilizes high-oxidation-state metals (e.g., Ti⁴⁺, Nd³⁺) by forming six-membered chelate rings. This enhances catalytic activity in oxidation and polymerization reactions. For example, neodymium complexes show high efficiency in stereospecific polymerizations due to their Lewis acidity and ligand lability .
Advanced Research Questions
Q. What experimental strategies can address contradictions in reaction yields for this compound-mediated catalysis?
- Methodological Answer : Contradictions often arise from solvent polarity, moisture sensitivity, or ligand-to-metal ratios. A systematic approach includes:
- Design of Experiments (DoE) : Vary parameters (temperature, solvent, stoichiometry) to identify optimal conditions .
- Kinetic Studies : Monitor reaction progress via in-situ IR or UV-Vis spectroscopy to detect intermediates .
- Control Experiments : Compare with analogous ligands (e.g., acetylacetonate derivatives) to isolate steric/electronic effects .
Q. How can computational modeling enhance the understanding of this compound’s electronic structure?
- Methodological Answer :
- DFT Calculations : Optimize geometry and compute frontier molecular orbitals (HOMO/LUMO) to predict reactivity .
- Molecular Dynamics (MD) : Simulate ligand substitution dynamics in solution to explain catalytic turnover rates .
- Charge Density Analysis : Use multipole refinement (via SHELXL) to map electron distribution in crystal structures .
Q. What role does this compound play in modulating stem cell pluripotency?
- Methodological Answer : The compound inhibits protein kinase D (PKD), which regulates cell differentiation pathways. Researchers use:
- In-Vitro Assays : Treat embryonic stem cells with controlled concentrations (1–10 µM) and assess pluripotency markers (Oct4, Nanog) via qPCR .
- Kinase Profiling : Screen against kinase libraries to confirm PKD selectivity .
- Dose-Response Studies : Establish EC₅₀ values and toxicity thresholds (e.g., via MTT assays) .
Properties
IUPAC Name |
4-oxopent-2-en-2-olate | |
---|---|---|
Details | Computed by LexiChem 2.6.6 (PubChem release 2019.06.18) | |
Source | PubChem | |
URL | https://pubchem.ncbi.nlm.nih.gov | |
Description | Data deposited in or computed by PubChem | |
InChI |
InChI=1S/C5H8O2/c1-4(6)3-5(2)7/h3,6H,1-2H3/p-1 | |
Details | Computed by InChI 1.0.5 (PubChem release 2019.06.18) | |
Source | PubChem | |
URL | https://pubchem.ncbi.nlm.nih.gov | |
Description | Data deposited in or computed by PubChem | |
InChI Key |
POILWHVDKZOXJZ-UHFFFAOYSA-M | |
Details | Computed by InChI 1.0.5 (PubChem release 2019.06.18) | |
Source | PubChem | |
URL | https://pubchem.ncbi.nlm.nih.gov | |
Description | Data deposited in or computed by PubChem | |
Canonical SMILES |
CC(=CC(=O)C)[O-] | |
Details | Computed by OEChem 2.1.5 (PubChem release 2019.06.18) | |
Source | PubChem | |
URL | https://pubchem.ncbi.nlm.nih.gov | |
Description | Data deposited in or computed by PubChem | |
Molecular Formula |
C5H7O2- | |
Details | Computed by PubChem 2.1 (PubChem release 2019.06.18) | |
Source | PubChem | |
URL | https://pubchem.ncbi.nlm.nih.gov | |
Description | Data deposited in or computed by PubChem | |
DSSTOX Substance ID |
DTXSID001293234 | |
Record name | 3-Penten-2-one, 4-hydroxy-, ion(1-) | |
Source | EPA DSSTox | |
URL | https://comptox.epa.gov/dashboard/DTXSID001293234 | |
Description | DSSTox provides a high quality public chemistry resource for supporting improved predictive toxicology. | |
Molecular Weight |
99.11 g/mol | |
Details | Computed by PubChem 2.1 (PubChem release 2021.05.07) | |
Source | PubChem | |
URL | https://pubchem.ncbi.nlm.nih.gov | |
Description | Data deposited in or computed by PubChem | |
CAS No. |
19268-49-6 | |
Record name | 3-Penten-2-one, 4-hydroxy-, ion(1-) | |
Source | CAS Common Chemistry | |
URL | https://commonchemistry.cas.org/detail?cas_rn=19268-49-6 | |
Description | CAS Common Chemistry is an open community resource for accessing chemical information. Nearly 500,000 chemical substances from CAS REGISTRY cover areas of community interest, including common and frequently regulated chemicals, and those relevant to high school and undergraduate chemistry classes. This chemical information, curated by our expert scientists, is provided in alignment with our mission as a division of the American Chemical Society. | |
Explanation | The data from CAS Common Chemistry is provided under a CC-BY-NC 4.0 license, unless otherwise stated. | |
Record name | 3-Penten-2-one, 4-hydroxy-, ion(1-) | |
Source | EPA DSSTox | |
URL | https://comptox.epa.gov/dashboard/DTXSID001293234 | |
Description | DSSTox provides a high quality public chemistry resource for supporting improved predictive toxicology. | |
Disclaimer and Information on In-Vitro Research Products
Please be aware that all articles and product information presented on BenchChem are intended solely for informational purposes. The products available for purchase on BenchChem are specifically designed for in-vitro studies, which are conducted outside of living organisms. In-vitro studies, derived from the Latin term "in glass," involve experiments performed in controlled laboratory settings using cells or tissues. It is important to note that these products are not categorized as medicines or drugs, and they have not received approval from the FDA for the prevention, treatment, or cure of any medical condition, ailment, or disease. We must emphasize that any form of bodily introduction of these products into humans or animals is strictly prohibited by law. It is essential to adhere to these guidelines to ensure compliance with legal and ethical standards in research and experimentation.