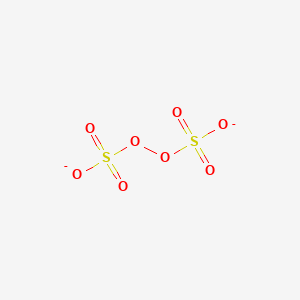
Peroxydisulfate
Overview
Description
Peroxydisulfate is a sulfur oxoanion and a sulfur oxide. It is a conjugate base of a peroxydisulfuric acid.
Scientific Research Applications
Water Treatment and Pollution Control
Peroxydisulfate (PDS) is effective in the degradation of pollutants in water. It's used in treating contaminated groundwater and industrial wastewaters. Activation of PDS, essential for its application due to its low reactivity, can be efficiently achieved with copper oxide (CuO) under mild conditions. This process avoids the generation of toxic halogenated products and is efficient at neutral pH, providing significant advantages over sulfate radical oxidation in the presence of chloride ions (Zhang et al., 2014). Additionally, activated PDS is used for the in situ chemical oxidation (ISCO) remediation of soil and groundwater, with changes in activation energy and kinetics in response to pH and temperature variations (Ma et al., 2017).
Activation for Contaminant Degradation
PDS can be activated by electrochemistry, using atomic hydrogen as an activator for the degradation of pollutants like norfloxacin over carbon-based nanocatalysts. This method has shown potential for practical applications in wastewater treatment (Yang et al., 2022). In another application, this compound activated by nanoscale zero-valent iron (nZVI) degrades sulfamethoxazole, an antibiotic frequently detected in the environment, in agricultural soils (Zhou et al., 2019).
Analytical and Measurement Techniques
In the realm of analytical chemistry, a method for the determination of this compound in purely aqueous solution has been developed, based on electrochemiluminescence with Cr(bpy)3^3+ (Xu & Dong, 2000). Similarly, ion chromatography with column-switching techniques has been utilized for the simultaneous analysis of this compound and conventional inorganic anions (Huang et al., 2014).
Chemical and Biological Processes
PDS also finds application in promoting the production of short-chain fatty acids and improving the properties of fermented sludge in waste treatment processes (Luo et al., 2019). Moreover, it's used in the degradation of various compounds like herbicides and pharmaceuticals, demonstrating its versatility in different environmental matrices (Diaz Kirmser et al., 2010).
Properties
CAS No. |
15092-81-6 |
---|---|
Molecular Formula |
O8S2-2 |
Molecular Weight |
192.13 g/mol |
IUPAC Name |
sulfonatooxy sulfate |
InChI |
InChI=1S/H2O8S2/c1-9(2,3)7-8-10(4,5)6/h(H,1,2,3)(H,4,5,6)/p-2 |
InChI Key |
JRKICGRDRMAZLK-UHFFFAOYSA-L |
SMILES |
[O-]S(=O)(=O)OOS(=O)(=O)[O-] |
Canonical SMILES |
[O-]S(=O)(=O)OOS(=O)(=O)[O-] |
Origin of Product |
United States |
Synthesis routes and methods
Procedure details
Retrosynthesis Analysis
AI-Powered Synthesis Planning: Our tool employs the Template_relevance Pistachio, Template_relevance Bkms_metabolic, Template_relevance Pistachio_ringbreaker, Template_relevance Reaxys, Template_relevance Reaxys_biocatalysis model, leveraging a vast database of chemical reactions to predict feasible synthetic routes.
One-Step Synthesis Focus: Specifically designed for one-step synthesis, it provides concise and direct routes for your target compounds, streamlining the synthesis process.
Accurate Predictions: Utilizing the extensive PISTACHIO, BKMS_METABOLIC, PISTACHIO_RINGBREAKER, REAXYS, REAXYS_BIOCATALYSIS database, our tool offers high-accuracy predictions, reflecting the latest in chemical research and data.
Strategy Settings
Precursor scoring | Relevance Heuristic |
---|---|
Min. plausibility | 0.01 |
Model | Template_relevance |
Template Set | Pistachio/Bkms_metabolic/Pistachio_ringbreaker/Reaxys/Reaxys_biocatalysis |
Top-N result to add to graph | 6 |
Feasible Synthetic Routes
Disclaimer and Information on In-Vitro Research Products
Please be aware that all articles and product information presented on BenchChem are intended solely for informational purposes. The products available for purchase on BenchChem are specifically designed for in-vitro studies, which are conducted outside of living organisms. In-vitro studies, derived from the Latin term "in glass," involve experiments performed in controlled laboratory settings using cells or tissues. It is important to note that these products are not categorized as medicines or drugs, and they have not received approval from the FDA for the prevention, treatment, or cure of any medical condition, ailment, or disease. We must emphasize that any form of bodily introduction of these products into humans or animals is strictly prohibited by law. It is essential to adhere to these guidelines to ensure compliance with legal and ethical standards in research and experimentation.