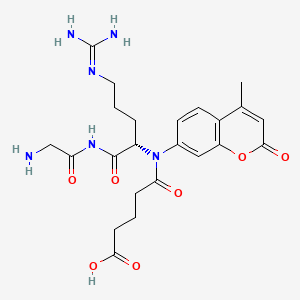
Glutaryl-glycyl-arginine-4-methylcoumaryl-7-amide
Overview
Description
Glutaryl-glycyl-arginine-4-methylcoumaryl-7-amide is a synthetic compound with a complex molecular structure. It is known for its applications in biochemical research, particularly as a fluorogenic substrate. The compound is characterized by its ability to emit fluorescence upon enzymatic cleavage, making it a valuable tool in various assays and diagnostic applications .
Preparation Methods
Synthetic Routes and Reaction Conditions: The synthesis of Glutaryl-glycyl-arginine-4-methylcoumaryl-7-amide involves multiple steps, starting with the preparation of the individual amino acid derivatives. The key steps include:
Coupling of Glutaryl and Glycyl: This step involves the formation of a peptide bond between glutaryl and glycyl using coupling reagents such as dicyclohexylcarbodiimide (DCC) and N-hydroxysuccinimide (NHS).
Addition of Arginine: The glycyl-glutaryl intermediate is then coupled with arginine using similar coupling reagents.
Attachment of 4-methylcoumaryl-7-amide: The final step involves the attachment of the 4-methylcoumaryl-7-amide moiety to the peptide chain, typically using a condensation reaction.
Industrial Production Methods: Industrial production of this compound follows similar synthetic routes but on a larger scale. The process is optimized for yield and purity, often involving automated peptide synthesizers and high-performance liquid chromatography (HPLC) for purification .
Types of Reactions:
Oxidation: The compound can undergo oxidation reactions, particularly at the arginine residue, leading to the formation of various oxidized derivatives.
Reduction: Reduction reactions can target the carbonyl groups present in the compound, converting them to alcohols.
Substitution: The aromatic ring of the 4-methylcoumaryl-7-amide moiety can undergo electrophilic substitution reactions.
Common Reagents and Conditions:
Oxidation: Common oxidizing agents include hydrogen peroxide and potassium permanganate.
Reduction: Reducing agents such as sodium borohydride and lithium aluminum hydride are used.
Substitution: Electrophilic substitution reactions often involve reagents like bromine or chlorine in the presence of a Lewis acid catalyst.
Major Products:
Oxidation Products: Oxidized derivatives of arginine and the coumaryl moiety.
Reduction Products: Alcohol derivatives of the carbonyl groups.
Substitution Products: Halogenated derivatives of the aromatic ring
Scientific Research Applications
Glutaryl-glycyl-arginine-4-methylcoumaryl-7-amide is widely used in scientific research due to its fluorogenic properties. Some key applications include:
Biochemical Assays: Used as a substrate in enzyme assays to measure the activity of proteolytic enzymes such as urokinase.
Diagnostic Tools: Employed in diagnostic assays to detect enzyme activity in clinical samples.
Drug Development: Utilized in high-throughput screening assays to identify potential inhibitors of proteolytic enzymes.
Molecular Biology: Applied in studies of protein-protein interactions and enzyme kinetics
Mechanism of Action
The mechanism of action of Glutaryl-glycyl-arginine-4-methylcoumaryl-7-amide involves its cleavage by specific proteolytic enzymes. Upon enzymatic cleavage, the compound releases the 4-methylcoumaryl-7-amide moiety, which emits fluorescence. This fluorescence can be quantitatively measured, providing insights into enzyme activity. The molecular targets include various proteolytic enzymes, and the pathways involved are primarily related to proteolysis and signal transduction .
Comparison with Similar Compounds
Glutaryl-glycyl-arginine-7-amido-4-methylcoumarin hydrochloride: A similar compound with a hydrochloride salt form, enhancing its solubility and stability.
Glutaryl-glycyl-arginine-4-methylcoumaryl-7-amide (free base): The non-salt form of the compound, used in different experimental conditions.
Uniqueness: this compound is unique due to its specific fluorogenic properties, making it highly valuable in enzyme assays. Its ability to emit fluorescence upon cleavage provides a sensitive and specific method for detecting proteolytic activity, distinguishing it from other similar compounds .
Properties
IUPAC Name |
5-[[(2S)-1-[(2-aminoacetyl)amino]-5-(diaminomethylideneamino)-1-oxopentan-2-yl]-(4-methyl-2-oxochromen-7-yl)amino]-5-oxopentanoic acid | |
---|---|---|
Source | PubChem | |
URL | https://pubchem.ncbi.nlm.nih.gov | |
Description | Data deposited in or computed by PubChem | |
InChI |
InChI=1S/C23H30N6O7/c1-13-10-21(34)36-17-11-14(7-8-15(13)17)29(19(31)5-2-6-20(32)33)16(4-3-9-27-23(25)26)22(35)28-18(30)12-24/h7-8,10-11,16H,2-6,9,12,24H2,1H3,(H,32,33)(H4,25,26,27)(H,28,30,35)/t16-/m0/s1 | |
Source | PubChem | |
URL | https://pubchem.ncbi.nlm.nih.gov | |
Description | Data deposited in or computed by PubChem | |
InChI Key |
UBMRGCAFOLVMCM-INIZCTEOSA-N | |
Source | PubChem | |
URL | https://pubchem.ncbi.nlm.nih.gov | |
Description | Data deposited in or computed by PubChem | |
Canonical SMILES |
CC1=CC(=O)OC2=C1C=CC(=C2)N(C(CCCN=C(N)N)C(=O)NC(=O)CN)C(=O)CCCC(=O)O | |
Source | PubChem | |
URL | https://pubchem.ncbi.nlm.nih.gov | |
Description | Data deposited in or computed by PubChem | |
Isomeric SMILES |
CC1=CC(=O)OC2=C1C=CC(=C2)N([C@@H](CCCN=C(N)N)C(=O)NC(=O)CN)C(=O)CCCC(=O)O | |
Source | PubChem | |
URL | https://pubchem.ncbi.nlm.nih.gov | |
Description | Data deposited in or computed by PubChem | |
Molecular Formula |
C23H30N6O7 | |
Source | PubChem | |
URL | https://pubchem.ncbi.nlm.nih.gov | |
Description | Data deposited in or computed by PubChem | |
DSSTOX Substance ID |
DTXSID10215461 | |
Record name | Glutaryl-glycyl-arginine-4-methylcoumaryl-7-amide | |
Source | EPA DSSTox | |
URL | https://comptox.epa.gov/dashboard/DTXSID10215461 | |
Description | DSSTox provides a high quality public chemistry resource for supporting improved predictive toxicology. | |
Molecular Weight |
502.5 g/mol | |
Source | PubChem | |
URL | https://pubchem.ncbi.nlm.nih.gov | |
Description | Data deposited in or computed by PubChem | |
CAS No. |
65147-16-2 | |
Record name | Glutaryl-glycyl-arginine-4-methylcoumaryl-7-amide | |
Source | ChemIDplus | |
URL | https://pubchem.ncbi.nlm.nih.gov/substance/?source=chemidplus&sourceid=0065147162 | |
Description | ChemIDplus is a free, web search system that provides access to the structure and nomenclature authority files used for the identification of chemical substances cited in National Library of Medicine (NLM) databases, including the TOXNET system. | |
Record name | Glutaryl-glycyl-arginine-4-methylcoumaryl-7-amide | |
Source | EPA DSSTox | |
URL | https://comptox.epa.gov/dashboard/DTXSID10215461 | |
Description | DSSTox provides a high quality public chemistry resource for supporting improved predictive toxicology. | |
Disclaimer and Information on In-Vitro Research Products
Please be aware that all articles and product information presented on BenchChem are intended solely for informational purposes. The products available for purchase on BenchChem are specifically designed for in-vitro studies, which are conducted outside of living organisms. In-vitro studies, derived from the Latin term "in glass," involve experiments performed in controlled laboratory settings using cells or tissues. It is important to note that these products are not categorized as medicines or drugs, and they have not received approval from the FDA for the prevention, treatment, or cure of any medical condition, ailment, or disease. We must emphasize that any form of bodily introduction of these products into humans or animals is strictly prohibited by law. It is essential to adhere to these guidelines to ensure compliance with legal and ethical standards in research and experimentation.