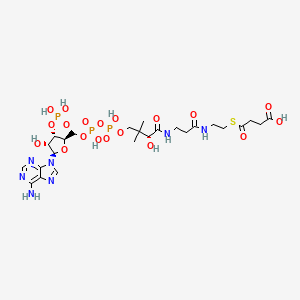
succinyl-CoA
Overview
Description
succinyl-CoA is a thioester of succinic acid and coenzyme A. It plays a crucial role as an intermediate in the citric acid cycle, where it is synthesized from α-ketoglutarate by α-ketoglutarate dehydrogenase through decarboxylation. This compound is essential for various metabolic pathways, including the synthesis of heme and the catabolism of certain amino acids and fatty acids .
Preparation Methods
Synthetic Routes and Reaction Conditions: succinyl-CoA can be synthesized by the addition of succinic anhydride to coenzyme A in the presence of a weak base . Another method involves the use of propionyl coenzyme A, which is carboxylated to D-methylmalonyl coenzyme A, isomerized to L-methylmalonyl coenzyme A, and rearranged to yield succinyl-coenzyme A via a vitamin B12-dependent enzyme .
Industrial Production Methods: Industrial production of succinyl-coenzyme A often involves microbial fermentation processes. Genetic engineering techniques are employed to enhance the production of coenzyme A derivatives in microorganisms, making the process more efficient and cost-effective .
Chemical Reactions Analysis
Types of Reactions: succinyl-CoA undergoes various chemical reactions, including:
Hydrolysis: It is converted into succinate through the hydrolytic release of coenzyme A by succinyl-coenzyme A synthetase.
Oxidation: In the citric acid cycle, succinyl-coenzyme A is oxidized to succinate, generating ATP or GTP in the process.
Common Reagents and Conditions:
Hydrolysis: Catalyzed by succinyl-coenzyme A synthetase.
Oxidation: Requires succinate dehydrogenase and occurs in the mitochondrial matrix.
Major Products:
Succinate: Formed through hydrolysis and oxidation reactions.
ATP or GTP: Generated during the conversion of succinyl-coenzyme A to succinate.
Scientific Research Applications
succinyl-CoA has diverse applications in scientific research:
Mechanism of Action
succinyl-CoA exerts its effects primarily through its role in the citric acid cycle. It is converted to succinate by succinyl-coenzyme A synthetase, generating ATP or GTP in the process. This conversion involves the displacement of coenzyme A by a nucleophilic inorganic phosphate molecule to form succinyl phosphate, followed by the generation of succinate and the transfer of the phosphate group to a nucleoside diphosphate .
Comparison with Similar Compounds
Acetyl-coenzyme A: Another thioester of coenzyme A, involved in the citric acid cycle and fatty acid metabolism.
Propionyl-coenzyme A: Involved in the catabolism of odd-chain fatty acids and certain amino acids.
Uniqueness: succinyl-CoA is unique due to its specific role in the citric acid cycle and its involvement in heme synthesis. Unlike acetyl-coenzyme A, which is primarily involved in fatty acid metabolism, succinyl-coenzyme A is crucial for the synthesis of succinate and the generation of ATP or GTP in the citric acid cycle .
Properties
IUPAC Name |
4-[2-[3-[[(2R)-4-[[[(2R,3S,4R,5R)-5-(6-aminopurin-9-yl)-4-hydroxy-3-phosphonooxyoxolan-2-yl]methoxy-hydroxyphosphoryl]oxy-hydroxyphosphoryl]oxy-2-hydroxy-3,3-dimethylbutanoyl]amino]propanoylamino]ethylsulfanyl]-4-oxobutanoic acid | |
---|---|---|
Source | PubChem | |
URL | https://pubchem.ncbi.nlm.nih.gov | |
Description | Data deposited in or computed by PubChem | |
InChI |
InChI=1S/C25H40N7O19P3S/c1-25(2,20(38)23(39)28-6-5-14(33)27-7-8-55-16(36)4-3-15(34)35)10-48-54(45,46)51-53(43,44)47-9-13-19(50-52(40,41)42)18(37)24(49-13)32-12-31-17-21(26)29-11-30-22(17)32/h11-13,18-20,24,37-38H,3-10H2,1-2H3,(H,27,33)(H,28,39)(H,34,35)(H,43,44)(H,45,46)(H2,26,29,30)(H2,40,41,42)/t13-,18-,19-,20+,24-/m1/s1 | |
Source | PubChem | |
URL | https://pubchem.ncbi.nlm.nih.gov | |
Description | Data deposited in or computed by PubChem | |
InChI Key |
VNOYUJKHFWYWIR-ITIYDSSPSA-N | |
Source | PubChem | |
URL | https://pubchem.ncbi.nlm.nih.gov | |
Description | Data deposited in or computed by PubChem | |
Canonical SMILES |
CC(C)(COP(=O)(O)OP(=O)(O)OCC1C(C(C(O1)N2C=NC3=C(N=CN=C32)N)O)OP(=O)(O)O)C(C(=O)NCCC(=O)NCCSC(=O)CCC(=O)O)O | |
Source | PubChem | |
URL | https://pubchem.ncbi.nlm.nih.gov | |
Description | Data deposited in or computed by PubChem | |
Isomeric SMILES |
CC(C)(COP(=O)(O)OP(=O)(O)OC[C@@H]1[C@H]([C@H]([C@@H](O1)N2C=NC3=C(N=CN=C32)N)O)OP(=O)(O)O)[C@H](C(=O)NCCC(=O)NCCSC(=O)CCC(=O)O)O | |
Source | PubChem | |
URL | https://pubchem.ncbi.nlm.nih.gov | |
Description | Data deposited in or computed by PubChem | |
Molecular Formula |
C25H40N7O19P3S | |
Source | PubChem | |
URL | https://pubchem.ncbi.nlm.nih.gov | |
Description | Data deposited in or computed by PubChem | |
DSSTOX Substance ID |
DTXSID40975809 | |
Record name | S-Succinoylcoenzyme A | |
Source | EPA DSSTox | |
URL | https://comptox.epa.gov/dashboard/DTXSID40975809 | |
Description | DSSTox provides a high quality public chemistry resource for supporting improved predictive toxicology. | |
Molecular Weight |
867.6 g/mol | |
Source | PubChem | |
URL | https://pubchem.ncbi.nlm.nih.gov | |
Description | Data deposited in or computed by PubChem | |
Physical Description |
Solid | |
Record name | Succinyl-CoA | |
Source | Human Metabolome Database (HMDB) | |
URL | http://www.hmdb.ca/metabolites/HMDB0001022 | |
Description | The Human Metabolome Database (HMDB) is a freely available electronic database containing detailed information about small molecule metabolites found in the human body. | |
Explanation | HMDB is offered to the public as a freely available resource. Use and re-distribution of the data, in whole or in part, for commercial purposes requires explicit permission of the authors and explicit acknowledgment of the source material (HMDB) and the original publication (see the HMDB citing page). We ask that users who download significant portions of the database cite the HMDB paper in any resulting publications. | |
CAS No. |
604-98-8 | |
Record name | Succinyl CoA | |
Source | CAS Common Chemistry | |
URL | https://commonchemistry.cas.org/detail?cas_rn=604-98-8 | |
Description | CAS Common Chemistry is an open community resource for accessing chemical information. Nearly 500,000 chemical substances from CAS REGISTRY cover areas of community interest, including common and frequently regulated chemicals, and those relevant to high school and undergraduate chemistry classes. This chemical information, curated by our expert scientists, is provided in alignment with our mission as a division of the American Chemical Society. | |
Explanation | The data from CAS Common Chemistry is provided under a CC-BY-NC 4.0 license, unless otherwise stated. | |
Record name | Succinyl-coenzyme A | |
Source | ChemIDplus | |
URL | https://pubchem.ncbi.nlm.nih.gov/substance/?source=chemidplus&sourceid=0000604988 | |
Description | ChemIDplus is a free, web search system that provides access to the structure and nomenclature authority files used for the identification of chemical substances cited in National Library of Medicine (NLM) databases, including the TOXNET system. | |
Record name | Succinyl-Coenzyme A | |
Source | DrugBank | |
URL | https://www.drugbank.ca/drugs/DB03699 | |
Description | The DrugBank database is a unique bioinformatics and cheminformatics resource that combines detailed drug (i.e. chemical, pharmacological and pharmaceutical) data with comprehensive drug target (i.e. sequence, structure, and pathway) information. | |
Explanation | Creative Common's Attribution-NonCommercial 4.0 International License (http://creativecommons.org/licenses/by-nc/4.0/legalcode) | |
Record name | S-Succinoylcoenzyme A | |
Source | EPA DSSTox | |
URL | https://comptox.epa.gov/dashboard/DTXSID40975809 | |
Description | DSSTox provides a high quality public chemistry resource for supporting improved predictive toxicology. | |
Record name | S-(hydrogen succinyl)coenzyme A | |
Source | European Chemicals Agency (ECHA) | |
URL | https://echa.europa.eu/substance-information/-/substanceinfo/100.009.163 | |
Description | The European Chemicals Agency (ECHA) is an agency of the European Union which is the driving force among regulatory authorities in implementing the EU's groundbreaking chemicals legislation for the benefit of human health and the environment as well as for innovation and competitiveness. | |
Explanation | Use of the information, documents and data from the ECHA website is subject to the terms and conditions of this Legal Notice, and subject to other binding limitations provided for under applicable law, the information, documents and data made available on the ECHA website may be reproduced, distributed and/or used, totally or in part, for non-commercial purposes provided that ECHA is acknowledged as the source: "Source: European Chemicals Agency, http://echa.europa.eu/". Such acknowledgement must be included in each copy of the material. ECHA permits and encourages organisations and individuals to create links to the ECHA website under the following cumulative conditions: Links can only be made to webpages that provide a link to the Legal Notice page. | |
Record name | SUCCINYL COENZYME A | |
Source | FDA Global Substance Registration System (GSRS) | |
URL | https://gsrs.ncats.nih.gov/ginas/app/beta/substances/BSI27HW5EQ | |
Description | The FDA Global Substance Registration System (GSRS) enables the efficient and accurate exchange of information on what substances are in regulated products. Instead of relying on names, which vary across regulatory domains, countries, and regions, the GSRS knowledge base makes it possible for substances to be defined by standardized, scientific descriptions. | |
Explanation | Unless otherwise noted, the contents of the FDA website (www.fda.gov), both text and graphics, are not copyrighted. They are in the public domain and may be republished, reprinted and otherwise used freely by anyone without the need to obtain permission from FDA. Credit to the U.S. Food and Drug Administration as the source is appreciated but not required. | |
Record name | Succinyl-CoA | |
Source | Human Metabolome Database (HMDB) | |
URL | http://www.hmdb.ca/metabolites/HMDB0001022 | |
Description | The Human Metabolome Database (HMDB) is a freely available electronic database containing detailed information about small molecule metabolites found in the human body. | |
Explanation | HMDB is offered to the public as a freely available resource. Use and re-distribution of the data, in whole or in part, for commercial purposes requires explicit permission of the authors and explicit acknowledgment of the source material (HMDB) and the original publication (see the HMDB citing page). We ask that users who download significant portions of the database cite the HMDB paper in any resulting publications. | |
Retrosynthesis Analysis
AI-Powered Synthesis Planning: Our tool employs the Template_relevance Pistachio, Template_relevance Bkms_metabolic, Template_relevance Pistachio_ringbreaker, Template_relevance Reaxys, Template_relevance Reaxys_biocatalysis model, leveraging a vast database of chemical reactions to predict feasible synthetic routes.
One-Step Synthesis Focus: Specifically designed for one-step synthesis, it provides concise and direct routes for your target compounds, streamlining the synthesis process.
Accurate Predictions: Utilizing the extensive PISTACHIO, BKMS_METABOLIC, PISTACHIO_RINGBREAKER, REAXYS, REAXYS_BIOCATALYSIS database, our tool offers high-accuracy predictions, reflecting the latest in chemical research and data.
Strategy Settings
Precursor scoring | Relevance Heuristic |
---|---|
Min. plausibility | 0.01 |
Model | Template_relevance |
Template Set | Pistachio/Bkms_metabolic/Pistachio_ringbreaker/Reaxys/Reaxys_biocatalysis |
Top-N result to add to graph | 6 |
Feasible Synthetic Routes
Disclaimer and Information on In-Vitro Research Products
Please be aware that all articles and product information presented on BenchChem are intended solely for informational purposes. The products available for purchase on BenchChem are specifically designed for in-vitro studies, which are conducted outside of living organisms. In-vitro studies, derived from the Latin term "in glass," involve experiments performed in controlled laboratory settings using cells or tissues. It is important to note that these products are not categorized as medicines or drugs, and they have not received approval from the FDA for the prevention, treatment, or cure of any medical condition, ailment, or disease. We must emphasize that any form of bodily introduction of these products into humans or animals is strictly prohibited by law. It is essential to adhere to these guidelines to ensure compliance with legal and ethical standards in research and experimentation.