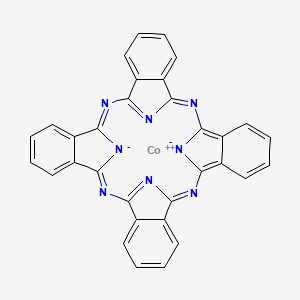
Cobalt phthalocyanin
Overview
Description
Phthalocyanine Cobalt(II) is a coordination compound with the formula C₃₂H₁₆CoN₈. It belongs to the phthalocyanine family, which are macrocyclic compounds structurally similar to porphyrins. Phthalocyanine Cobalt(II) is known for its intense blue-green color and is widely used in various applications due to its stability, electronic properties, and catalytic activity .
Mechanism of Action
Target of Action
Cobalt Phthalocyanine (CoPc) primarily targets the high-valent cobalt species . These species have been identified as highly efficient active centers for the oxygen evolution reaction (OER) . The OER is a crucial process in various energy conversion technologies, including water splitting and rechargeable metal-air batteries .
Mode of Action
CoPc interacts with its targets by accelerating the formation of high-valent cobalt species under OER conditions . This is achieved by decorating a π-conjugated molecule (CoPc) on Co-MOF-74 . The strong electronic interaction between Co-MOF-74 and CoPc is revealed by XPS and Raman characterizations . Furthermore, CoPc can promote the transformation of Co-MOF-74 to α-Co(OH)2 species under low potential, which then facilitates the generation of disordered CoOOH and Co(IV)O2 species during the water oxidation process .
Biochemical Pathways
The primary biochemical pathway affected by CoPc is the oxygen evolution reaction (OER) . CoPc accelerates the formation of high-valent cobalt species, which are the active centers for OER . This leads to enhanced kinetics for the formation of the O–O bond . The transformation of Co-MOF-74 to α-Co(OH)2 species under low potential, facilitated by CoPc, is a crucial step in this pathway .
Pharmacokinetics
The local coordination environment of CoPc plays a significant role in this process .
Result of Action
The action of CoPc results in the generation of high-valent cobalt species, which are highly efficient active centers for the oxygen evolution reaction . This leads to enhanced kinetics for the formation of the O–O bond . The obtained Co-MOF-74@CoPc displays a low overpotential and robust long-term stability .
Action Environment
The action, efficacy, and stability of CoPc can be influenced by environmental factors. For instance, the generation of high-valent cobalt species is thermodynamically unfavorable under OER conditions . The introduction of copc can overcome this challenge and accelerate the formation of these species . Furthermore, the local coordination environment of CoPc, regulated by introducing either electron-donating or electron-withdrawing groups on the phthalocyanine ligand, can also influence its action .
Biochemical Analysis
Biochemical Properties
Cobalt phthalocyanin plays a crucial role in biochemical reactions, particularly in electrocatalysis and redox reactions. It interacts with various enzymes, proteins, and biomolecules, facilitating electron transfer processes. For instance, this compound has been shown to interact with cytochrome P450 enzymes, enhancing their catalytic activity in oxidation reactions . Additionally, it can bind to proteins such as hemoglobin, altering their redox states and influencing oxygen transport and storage . These interactions are primarily driven by the coordination of the cobalt ion with the active sites of the enzymes and proteins, leading to changes in their electronic and structural properties.
Cellular Effects
This compound exerts significant effects on various types of cells and cellular processes. It influences cell function by modulating cell signaling pathways, gene expression, and cellular metabolism. For example, this compound has been found to activate the hypoxia-inducible factor (HIF) pathway, leading to increased expression of genes involved in angiogenesis and erythropoiesis . Furthermore, it can affect cellular metabolism by altering the activity of key metabolic enzymes, such as lactate dehydrogenase and pyruvate kinase, thereby influencing glycolysis and oxidative phosphorylation . These effects highlight the potential of this compound as a modulator of cellular functions and a therapeutic agent.
Molecular Mechanism
The molecular mechanism of action of this compound involves its ability to bind to biomolecules, inhibit or activate enzymes, and modulate gene expression. At the molecular level, this compound can coordinate with the active sites of enzymes, leading to changes in their catalytic activity. For instance, it has been shown to inhibit the activity of acetylcholinesterase by binding to its active site, thereby affecting neurotransmission . Additionally, this compound can interact with DNA and RNA, influencing gene expression by modulating transcription and translation processes . These molecular interactions underpin the diverse biochemical effects of this compound.
Temporal Effects in Laboratory Settings
In laboratory settings, the effects of this compound can change over time due to its stability, degradation, and long-term impact on cellular function. Studies have shown that this compound is relatively stable under physiological conditions, maintaining its catalytic activity over extended periods . It can undergo degradation in the presence of strong oxidizing agents, leading to the formation of inactive by-products . Long-term exposure to this compound has been associated with changes in cellular function, including alterations in cell proliferation, differentiation, and apoptosis . These temporal effects are crucial for understanding the potential therapeutic and toxicological implications of this compound.
Dosage Effects in Animal Models
The effects of this compound vary with different dosages in animal models. At low doses, this compound has been shown to enhance antioxidant defenses and protect against oxidative stress . At high doses, it can induce toxic effects, including hepatotoxicity, nephrotoxicity, and neurotoxicity . These adverse effects are likely due to the accumulation of cobalt ions in tissues, leading to oxidative damage and disruption of cellular homeostasis . Understanding the dosage-dependent effects of this compound is essential for its safe and effective use in therapeutic applications.
Metabolic Pathways
This compound is involved in various metabolic pathways, interacting with enzymes and cofactors to modulate metabolic flux and metabolite levels. It has been shown to participate in the tricarboxylic acid (TCA) cycle by influencing the activity of key enzymes such as citrate synthase and succinate dehydrogenase . Additionally, this compound can affect the pentose phosphate pathway by modulating the activity of glucose-6-phosphate dehydrogenase, thereby influencing the production of NADPH and ribose-5-phosphate . These interactions highlight the role of this compound in regulating cellular metabolism and energy production.
Transport and Distribution
The transport and distribution of this compound within cells and tissues are mediated by various transporters and binding proteins. It can be transported across cell membranes via metal ion transporters such as divalent metal transporter 1 (DMT1) and zinc transporter (ZnT) proteins . Once inside the cell, this compound can bind to intracellular proteins such as metallothioneins and ferritins, influencing its localization and accumulation . These transport and distribution mechanisms are critical for understanding the bioavailability and cellular effects of this compound.
Subcellular Localization
This compound exhibits specific subcellular localization, which can affect its activity and function. It has been found to localize in mitochondria, where it can influence mitochondrial respiration and reactive oxygen species (ROS) production . Additionally, this compound can be targeted to the nucleus, where it can interact with DNA and modulate gene expression . These subcellular localization patterns are determined by targeting signals and post-translational modifications that direct this compound to specific compartments or organelles . Understanding the subcellular localization of this compound is essential for elucidating its biochemical and cellular effects.
Preparation Methods
Synthetic Routes and Reaction Conditions: Phthalocyanine Cobalt(II) can be synthesized through several methods. One common method involves the reaction of phthalonitrile with cobalt chloride in the presence of a base such as ammonium molybdate and urea. The reaction is typically carried out in a high-boiling solvent like nitrobenzene at elevated temperatures . Another method involves heating phthalonitrile with cobalt chloride in the presence of a catalyst such as ammonium chloride and urea .
Industrial Production Methods: Industrial production of Phthalocyanine Cobalt(II) often involves similar synthetic routes but on a larger scale. The reaction conditions are optimized to ensure high yield and purity. The product is then purified through recrystallization or other separation techniques to obtain the desired compound .
Chemical Reactions Analysis
Types of Reactions: Phthalocyanine Cobalt(II) undergoes various chemical reactions, including oxidation, reduction, and substitution reactions. It is particularly known for its catalytic activity in oxidation reactions .
Common Reagents and Conditions:
Oxidation: Phthalocyanine Cobalt(II) can catalyze the oxidation of phenolic compounds to quinones and aldehydes.
Substitution: Substitution reactions can occur on the phthalocyanine ring, where various substituents can be introduced to modify its properties.
Major Products:
Scientific Research Applications
Phthalocyanine Cobalt(II) has a wide range of applications in scientific research:
Comparison with Similar Compounds
Phthalocyanine Cobalt(II) can be compared with other metal phthalocyanines, such as those containing iron, copper, and nickel. Each of these compounds has unique properties and catalytic activities:
Phthalocyanine Iron(II): Known for its catalytic activity in oxidation reactions, particularly in the oxidation of hydrocarbons.
Phthalocyanine Copper(II): Widely used in dye and pigment production due to its intense blue color.
Phthalocyanine Nickel(II): Used in electrocatalysis and as a catalyst in various organic reactions.
Phthalocyanine Cobalt(II) is unique in its ability to catalyze both oxidation and reduction reactions effectively, making it a versatile compound in various applications .
Properties
CAS No. |
3317-67-7 |
---|---|
Molecular Formula |
C32H16CoN8 |
Molecular Weight |
571.5 g/mol |
IUPAC Name |
cobalt(2+);2,11,20,29,37,39-hexaza-38,40-diazanidanonacyclo[28.6.1.13,10.112,19.121,28.04,9.013,18.022,27.031,36]tetraconta-1,3,5,7,9,11,13,15,17,19(39),20,22,24,26,28,30(37),31,33,35-nonadecaene |
InChI |
InChI=1S/C32H16N8.Co/c1-2-10-18-17(9-1)25-33-26(18)38-28-21-13-5-6-14-22(21)30(35-28)40-32-24-16-8-7-15-23(24)31(36-32)39-29-20-12-4-3-11-19(20)27(34-29)37-25;/h1-16H;/q-2;+2 |
InChI Key |
MPMSMUBQXQALQI-UHFFFAOYSA-N |
SMILES |
C1=CC=C2C(=C1)C3=NC4=NC(=NC5=C6C=CC=CC6=C([N-]5)N=C7C8=CC=CC=C8C(=N7)N=C2[N-]3)C9=CC=CC=C94.[Co+2] |
Canonical SMILES |
C1=CC=C2C(=C1)C3=NC4=NC(=NC5=C6C=CC=CC6=C([N-]5)N=C7C8=CC=CC=C8C(=N7)N=C2[N-]3)C9=CC=CC=C94.[Co+2] |
Key on ui other cas no. |
3317-67-7 |
physical_description |
Dark odorless powder; [Alfa Aesar MSDS] |
Pictograms |
Health Hazard |
Related CAS |
36344-62-4 |
Synonyms |
cobalt phthalocyanine |
Origin of Product |
United States |
Retrosynthesis Analysis
AI-Powered Synthesis Planning: Our tool employs the Template_relevance Pistachio, Template_relevance Bkms_metabolic, Template_relevance Pistachio_ringbreaker, Template_relevance Reaxys, Template_relevance Reaxys_biocatalysis model, leveraging a vast database of chemical reactions to predict feasible synthetic routes.
One-Step Synthesis Focus: Specifically designed for one-step synthesis, it provides concise and direct routes for your target compounds, streamlining the synthesis process.
Accurate Predictions: Utilizing the extensive PISTACHIO, BKMS_METABOLIC, PISTACHIO_RINGBREAKER, REAXYS, REAXYS_BIOCATALYSIS database, our tool offers high-accuracy predictions, reflecting the latest in chemical research and data.
Strategy Settings
Precursor scoring | Relevance Heuristic |
---|---|
Min. plausibility | 0.01 |
Model | Template_relevance |
Template Set | Pistachio/Bkms_metabolic/Pistachio_ringbreaker/Reaxys/Reaxys_biocatalysis |
Top-N result to add to graph | 6 |
Feasible Synthetic Routes
Disclaimer and Information on In-Vitro Research Products
Please be aware that all articles and product information presented on BenchChem are intended solely for informational purposes. The products available for purchase on BenchChem are specifically designed for in-vitro studies, which are conducted outside of living organisms. In-vitro studies, derived from the Latin term "in glass," involve experiments performed in controlled laboratory settings using cells or tissues. It is important to note that these products are not categorized as medicines or drugs, and they have not received approval from the FDA for the prevention, treatment, or cure of any medical condition, ailment, or disease. We must emphasize that any form of bodily introduction of these products into humans or animals is strictly prohibited by law. It is essential to adhere to these guidelines to ensure compliance with legal and ethical standards in research and experimentation.