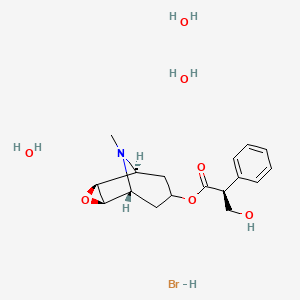
Scopolamine hydrobromide trihydrate
Overview
Description
Mechanism of Action
Target of Action
Scopolamine hydrobromide trihydrate primarily targets the muscarinic acetylcholine receptors (mAChRs) . These receptors are found in the central nervous system and throughout the body, playing a crucial role in the parasympathetic nervous system and cholinergic signaling .
Mode of Action
This compound acts as a competitive antagonist at muscarinic acetylcholine receptors . This means it competes with the neurotransmitter acetylcholine for the same binding sites on the mAChRs . By binding to these receptors, this compound inhibits the action of acetylcholine, leading to several therapeutic and adverse effects related to the alteration of the parasympathetic nervous system and cholinergic signaling .
Biochemical Pathways
The biochemical pathways affected by this compound are those involving the neurotransmitter acetylcholine and its interaction with muscarinic acetylcholine receptors . By antagonizing these receptors, this compound disrupts the normal functioning of the parasympathetic nervous system, which can lead to various physiological effects .
Pharmacokinetics
The pharmacokinetics of this compound involve its absorption, distribution, metabolism, and excretion (ADME). It has a bioavailability of 20-40% and is metabolized in the liver by the enzyme CYP3A4 . The elimination half-life of this compound is approximately 5 hours , and it is excreted through the kidneys .
Result of Action
The molecular and cellular effects of this compound’s action include a decrease in the activity of the parasympathetic nervous system and a disruption of cholinergic signaling . This can lead to various therapeutic effects, such as the prevention of nausea and vomiting associated with motion sickness and postoperative procedures . It can also lead to adverse effects, which are dose-dependent .
Biochemical Analysis
Biochemical Properties
Scopolamine hydrobromide trihydrate acts as a competitive nonselective muscarinic acetylcholine antagonist. It interacts with muscarinic acetylcholine receptors (mAChRs) in the central nervous system and throughout the body. By binding to these receptors, it inhibits the action of acetylcholine, a neurotransmitter involved in various physiological functions. This interaction leads to the inhibition of parasympathetic nerve impulses, resulting in effects such as reduced saliva and sweat secretion, decreased gastrointestinal motility, and pupil dilation .
Cellular Effects
This compound influences various cellular processes by blocking muscarinic receptors. In neurons, it inhibits acetylcholine-mediated signaling, leading to decreased neurotransmission and altered cognitive functions. This compound is often used to induce amnesia in laboratory animals, serving as a model for memory deficits. Additionally, this compound affects cell signaling pathways, gene expression, and cellular metabolism by modulating the activity of mAChRs .
Molecular Mechanism
At the molecular level, this compound exerts its effects by binding to muscarinic acetylcholine receptors. This binding prevents acetylcholine from activating these receptors, thereby inhibiting downstream signaling pathways. The compound’s anticholinergic activity results in the inhibition of enzyme activities and changes in gene expression related to cholinergic signaling. This mechanism underlies its therapeutic effects in treating motion sickness and postoperative nausea .
Temporal Effects in Laboratory Settings
In laboratory settings, the effects of this compound can vary over time. The compound is known to be stable under controlled conditions but may degrade when exposed to light and air. Long-term studies have shown that this compound can induce persistent changes in cellular function, including alterations in neurotransmitter levels and receptor sensitivity. These temporal effects are crucial for understanding its long-term impact on cellular processes .
Dosage Effects in Animal Models
The effects of this compound in animal models are dose-dependent. At low doses, it can induce mild anticholinergic effects, such as reduced salivation and pupil dilation. Higher doses can lead to more pronounced effects, including severe cognitive impairment, altered locomotor activity, and anxiety-like behaviors. Toxic effects, such as respiratory depression and convulsions, have been observed at very high doses .
Metabolic Pathways
This compound is metabolized primarily in the liver by the enzyme cytochrome P450 3A4 (CYP3A4). The metabolic pathways involve the hydroxylation and conjugation of the compound, leading to the formation of inactive metabolites that are excreted via the kidneys. This metabolism affects the compound’s bioavailability and duration of action .
Transport and Distribution
This compound is transported and distributed within the body through various mechanisms. It can cross the blood-brain barrier, allowing it to exert central nervous system effects. The compound is also distributed to peripheral tissues, where it interacts with muscarinic receptors. Its distribution is influenced by factors such as lipid solubility and protein binding .
Subcellular Localization
Within cells, this compound is localized primarily in the cytoplasm and can interact with intracellular muscarinic receptors. It may also be found in other subcellular compartments, such as the endoplasmic reticulum and Golgi apparatus, where it can influence receptor trafficking and signaling. The compound’s subcellular localization is critical for its pharmacological activity and therapeutic effects .
Preparation Methods
Synthetic Routes and Reaction Conditions
Scopolamine hydrobromide can be synthesized through various methods. One efficient method involves the magnetic field-induced crystallization of scopolamine. This process leverages the difference in solubility between scopolamine and scopolamine hydrobromide, followed by salifying crystallization under the influence of a magnetic field. This method enhances the purity and recovery rate of the compound .
Industrial Production Methods
Industrial production of scopolamine hydrobromide primarily relies on the large-scale cultivation of hybrid plants, such as Duboisia myoporoides and Duboisia leichhardtii. These plants are rich in tropane alkaloids, including scopolamine. The alkaloids are extracted and purified to produce scopolamine hydrobromide .
Chemical Reactions Analysis
Types of Reactions
Scopolamine hydrobromide undergoes various chemical reactions, including:
Oxidation: Scopolamine can be oxidized to form scopolamine N-oxide.
Reduction: Reduction reactions can convert scopolamine to its corresponding alcohol derivatives.
Substitution: Scopolamine can undergo substitution reactions, particularly at the ester and amine functional groups.
Common Reagents and Conditions
Oxidation: Common oxidizing agents include hydrogen peroxide and potassium permanganate.
Reduction: Reducing agents such as lithium aluminum hydride and sodium borohydride are used.
Substitution: Substitution reactions often involve reagents like alkyl halides and acyl chlorides.
Major Products
Oxidation: Scopolamine N-oxide.
Reduction: Alcohol derivatives of scopolamine.
Substitution: Various substituted scopolamine derivatives, depending on the reagents used.
Scientific Research Applications
Scopolamine hydrobromide has a wide range of scientific research applications:
Chemistry: Used as a substrate for synthesizing other compounds, such as tiotropium bromide.
Biology: Employed in studies investigating the effects of anticholinergic agents on biological systems.
Medicine: Utilized in clinical research to explore its efficacy in treating motion sickness, postoperative nausea, and other conditions.
Industry: Applied in the production of pharmaceuticals and as a research tool in drug development
Comparison with Similar Compounds
Similar Compounds
Atropine: Another tropane alkaloid with similar anticholinergic properties.
Hyoscyamine: Structurally similar to scopolamine, with comparable pharmacological effects.
Tiotropium Bromide: A synthetic derivative used in the treatment of chronic obstructive pulmonary disease (COPD).
Uniqueness
Scopolamine hydrobromide is unique due to its higher physiological activity and fewer adverse effects compared to hyoscyamine. It is also a more potent mydriatic and suppressant of salivation than hyoscyamine, making it particularly valuable in medical applications .
Properties
CAS No. |
6533-68-2 |
---|---|
Molecular Formula |
C17H21NO4.BrH.3H2O C17H28BrNO7 |
Molecular Weight |
438.3 g/mol |
IUPAC Name |
[(1S,2S,4R,5R)-9-methyl-3-oxa-9-azatricyclo[3.3.1.02,4]nonan-7-yl] 3-hydroxy-2-phenylpropanoate;trihydrate;hydrobromide |
InChI |
InChI=1S/C17H21NO4.BrH.3H2O/c1-18-13-7-11(8-14(18)16-15(13)22-16)21-17(20)12(9-19)10-5-3-2-4-6-10;;;;/h2-6,11-16,19H,7-9H2,1H3;1H;3*1H2/t11?,12?,13-,14+,15-,16+;;;; |
InChI Key |
LACQPOBCQQPVIT-YXJJTENCSA-N |
Isomeric SMILES |
CN1[C@@H]2CC(C[C@H]1[C@H]3[C@@H]2O3)OC(=O)C(CO)C4=CC=CC=C4.O.O.O.Br |
SMILES |
CN1C2CC(CC1C3C2O3)OC(=O)C(CO)C4=CC=CC=C4.O.O.O.Br |
Canonical SMILES |
CN1C2CC(CC1C3C2O3)OC(=O)C(CO)C4=CC=CC=C4.O.O.O.Br |
melting_point |
131 °F (NTP, 1992) |
6533-68-2 | |
physical_description |
Scopolamine hydrobromide trihydrate appears as white orthorhombic sphenoidal crystals from water. pH of 0.05 molar solution: 5.85. (NTP, 1992) |
Pictograms |
Acute Toxic |
solubility |
1 gm/1.5 mL (NTP, 1992) |
Origin of Product |
United States |
Retrosynthesis Analysis
AI-Powered Synthesis Planning: Our tool employs the Template_relevance Pistachio, Template_relevance Bkms_metabolic, Template_relevance Pistachio_ringbreaker, Template_relevance Reaxys, Template_relevance Reaxys_biocatalysis model, leveraging a vast database of chemical reactions to predict feasible synthetic routes.
One-Step Synthesis Focus: Specifically designed for one-step synthesis, it provides concise and direct routes for your target compounds, streamlining the synthesis process.
Accurate Predictions: Utilizing the extensive PISTACHIO, BKMS_METABOLIC, PISTACHIO_RINGBREAKER, REAXYS, REAXYS_BIOCATALYSIS database, our tool offers high-accuracy predictions, reflecting the latest in chemical research and data.
Strategy Settings
Precursor scoring | Relevance Heuristic |
---|---|
Min. plausibility | 0.01 |
Model | Template_relevance |
Template Set | Pistachio/Bkms_metabolic/Pistachio_ringbreaker/Reaxys/Reaxys_biocatalysis |
Top-N result to add to graph | 6 |
Feasible Synthetic Routes
Disclaimer and Information on In-Vitro Research Products
Please be aware that all articles and product information presented on BenchChem are intended solely for informational purposes. The products available for purchase on BenchChem are specifically designed for in-vitro studies, which are conducted outside of living organisms. In-vitro studies, derived from the Latin term "in glass," involve experiments performed in controlled laboratory settings using cells or tissues. It is important to note that these products are not categorized as medicines or drugs, and they have not received approval from the FDA for the prevention, treatment, or cure of any medical condition, ailment, or disease. We must emphasize that any form of bodily introduction of these products into humans or animals is strictly prohibited by law. It is essential to adhere to these guidelines to ensure compliance with legal and ethical standards in research and experimentation.