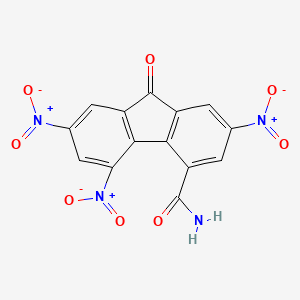
2,5,7-Trinitro-9-oxofluorene-4-carboxamide
- Click on QUICK INQUIRY to receive a quote from our team of experts.
- With the quality product at a COMPETITIVE price, you can focus more on your research.
Overview
Description
2,5,7-Trinitro-9-oxofluorene-4-carboxamide is a polyfunctional fluorene derivative characterized by three nitro (-NO₂) groups at positions 2, 5, and 7, a ketone (oxo) group at position 9, and a carboxamide (-CONH₂) group at position 3.
Preparation Methods
Synthetic Routes and Reaction Conditions
The synthesis of 2,5,7-Trinitro-9-oxofluorene-4-carboxamide typically involves the nitration of fluorene derivatives. One common method is the nitration of 9-fluorenone-4-carboxylic acid, followed by the conversion of the resulting trinitro compound to the carboxamide. The reaction conditions often include the use of concentrated nitric acid and sulfuric acid as nitrating agents, with careful temperature control to avoid over-nitration and decomposition of the product .
Industrial Production Methods
While specific industrial production methods for this compound are not well-documented, the general approach would involve scaling up the laboratory synthesis methods. This would include optimizing reaction conditions for higher yields and purity, as well as implementing safety measures to handle the highly reactive and potentially hazardous nitrating agents.
Chemical Reactions Analysis
Types of Reactions
2,5,7-Trinitro-9-oxofluorene-4-carboxamide undergoes various chemical reactions, including:
Oxidation: The compound can be further oxidized under strong oxidizing conditions, potentially leading to the formation of more highly oxidized derivatives.
Reduction: Reduction of the nitro groups can be achieved using reducing agents such as hydrogen gas in the presence of a catalyst, or chemical reductants like tin(II) chloride.
Substitution: The nitro groups can be substituted by nucleophiles under appropriate conditions, leading to the formation of different substituted derivatives
Common Reagents and Conditions
Oxidation: Strong oxidizing agents like potassium permanganate or chromium trioxide.
Reduction: Hydrogen gas with a palladium catalyst, or chemical reductants like tin(II) chloride.
Substitution: Nucleophiles such as amines or thiols in the presence of a base.
Major Products Formed
The major products formed from these reactions depend on the specific conditions and reagents used. For example, reduction of the nitro groups can lead to the formation of amino derivatives, while substitution reactions can yield a variety of substituted fluorene compounds .
Scientific Research Applications
Medicinal Chemistry
2,5,7-Trinitro-9-oxofluorene-4-carboxamide has shown potential as a pharmacophore in drug development:
- Antimicrobial Activity : Studies have indicated that this compound exhibits significant antibacterial properties against pathogens like Staphylococcus aureus and Escherichia coli, with mechanisms involving disruption of bacterial respiratory functions .
- Antioxidant Properties : In vitro assays demonstrated its ability to scavenge free radicals and inhibit lipid peroxidation, suggesting protective effects against oxidative stress .
Materials Science
The compound's unique structural properties make it valuable in developing advanced materials:
- Graphene-Based Composites : Research has shown that this compound can be used to synthesize graphene-based inks with enhanced electrical properties. These materials are promising for applications in flexible electronics .
Environmental Studies
The environmental impact of nitro compounds is significant due to their potential toxicity and persistence:
- Genotoxicity Studies : Research indicates that derivatives of this compound can exhibit mutagenic effects in microbial assays. Understanding these effects is crucial for assessing environmental risks associated with nitro compounds .
Case Studies
- Antimicrobial Agents : A study demonstrated that derivatives of this compound exhibited enhanced antibacterial activity compared to traditional antibiotics. This highlights its potential as a lead compound for new antimicrobial therapies .
- Cancer Treatment : Preliminary investigations suggest that the compound may induce apoptosis in cancer cell lines through mechanisms involving oxidative stress responses. This positions it as a candidate for further research in oncology .
- Graphene Ink Development : The synthesis of a novel graphene-based material using this compound has been reported to yield inks suitable for printed electronics, showcasing its versatility beyond traditional chemical applications .
Mechanism of Action
The mechanism of action of 2,5,7-Trinitro-9-oxofluorene-4-carboxamide involves its interaction with molecular targets through its nitro and carboxamide groups. These functional groups can participate in various chemical interactions, including hydrogen bonding, electron transfer, and nucleophilic substitution. The specific pathways and molecular targets depend on the context of its application, such as its use in biological systems or chemical reactions .
Comparison with Similar Compounds
Comparison with Structurally Similar Compounds
The following analysis compares 2,5,7-Trinitro-9-oxofluorene-4-carboxamide with two closely related fluorene derivatives, highlighting functional group contributions, molecular properties, and research findings.
Table 1: Key Molecular Properties of Comparable Fluorene Derivatives
*†Inferred formula based on structural analogy.
Functional Group Analysis
Nitro Groups: The three nitro groups in 2,5,7-Trinitro-9H-fluorene-4-carboxylic acid () contribute to its high molecular weight (345.223 g/mol) and likely explosive properties due to nitro’s oxidizing nature .
Oxo (Ketone) Group :
- The oxo group at position 9 in 9-oxo-9H-fluorene-4-carboxamide () introduces electron-withdrawing effects, stabilizing the aromatic system and enhancing intermolecular interactions in biological systems .
- In the target compound, this group may similarly modulate electronic properties, affecting reactivity in substitution or redox reactions.
Carboxamide vs. Carboxylic Acid: The carboxamide group in ’s compound (C₁₄H₉NO₂) reduces acidity compared to the carboxylic acid in (pKa ~2–3 vs. ~4–5 for carboxamide). This increases solubility in polar aprotic solvents and enhances hydrogen-bonding capacity, making it more suitable for biological applications . The target compound’s carboxamide group would likely confer similar advantages, such as improved bioavailability if used pharmaceutically.
Stability and Reactivity
- Thermal Stability :
’s trinitro-carboxylic acid derivative is expected to exhibit low thermal stability due to nitro groups, a trait shared with the target compound. In contrast, ’s carboxamide lacks nitro groups, resulting in higher thermal stability (as indicated by its use in crystallography studies) . - Aromatic System : Both and the target compound feature a fluorene backbone with extended conjugation. However, the nitro groups in the target compound would reduce electron density, increasing susceptibility to nucleophilic attack compared to ’s carboxamide .
Biological Activity
2,5,7-Trinitro-9-oxofluorene-4-carboxamide (TNF-COOH) is a complex organic compound characterized by its unique molecular structure, which includes multiple nitro groups and a carboxamide functional group. This compound has garnered attention for its potential biological activities and applications in medicinal chemistry and materials science.
The molecular formula of this compound is C14H6N4O8. Its structure features three nitro groups attached to the fluorene skeleton, significantly enhancing its reactivity compared to similar compounds. The presence of the carboxamide group contributes to its biological interactions.
Property | Value |
---|---|
Molecular Formula | C14H6N4O8 |
Molecular Weight | 342.22 g/mol |
Melting Point | 267–269 °C |
Solubility | Insoluble in water |
The biological activity of TNF-COOH is attributed to its interactions with various biomolecules and cellular pathways. Research indicates that it may induce cytotoxic effects and exhibit mutagenic properties. The compound has been shown to interact with proteins involved in cell signaling and gene regulation.
Target Interactions
- Cytotoxicity : TNF-COOH has demonstrated cytotoxic effects on various cell lines, indicating potential applications in cancer therapy.
- Genotoxicity : Studies have reported that TNF-COOH induces mutations in prokaryotic and eukaryotic cells, suggesting its role as a mutagenic agent .
Biological Activity Studies
Several studies have explored the biological activity of TNF-COOH, focusing on its effects on different cell types and mechanisms.
Case Study: Genotoxic Effects
A study published in PubMed investigated the genotoxic properties of TNF-COOH using assays on Salmonella typhimurium and Escherichia coli. The findings revealed that TNF-COOH is a potent mutagen, causing both frame-shift and base-pair substitution mutations . In Saccharomyces cerevisiae, the compound's toxicity hindered the detection of genotoxic effects.
Cytotoxic Activity
Another study assessed the cytotoxic effects of TNF-COOH on mouse lymphoma cells and Chinese hamster ovary (CHO) cells. The results indicated that metabolic activation reduced toxicity, highlighting the importance of metabolic pathways in modulating the compound's effects .
Applications in Medicinal Chemistry
The unique properties of TNF-COOH make it a candidate for various applications:
Q & A
Basic Research Questions
Q. What methodological approaches are recommended for synthesizing 2,5,7-Trinitro-9-oxofluorene-4-carboxamide with high purity?
- Answer : Utilize stepwise nitration and carboxamide functionalization under controlled conditions (e.g., low temperature for nitro-group stability). Monitor reaction progress via TLC and HPLC. Purification can involve recrystallization from polar aprotic solvents, validated by melting point consistency (e.g., mp ranges for analogous nitro compounds: 113–124°C ). Purity should be confirmed via ≥95% HPLC and NMR integration .
Q. How can researchers validate the structural integrity of this compound post-synthesis?
- Answer : Combine spectroscopic techniques:
- FT-IR for nitro (∼1520 cm⁻¹) and carbonyl (∼1680 cm⁻¹) groups.
- ¹H/¹³C NMR to confirm aromatic proton environments and carboxamide resonance.
- Mass spectrometry (HRMS) for molecular ion alignment with theoretical m/z.
Cross-referencing with computational predictions (e.g., DFT for spectral simulation) reduces ambiguity .
Q. What are best practices for storing this compound to prevent degradation?
- Answer : Store in airtight, light-resistant containers at 0–6°C, as recommended for thermally sensitive nitroaromatics . Conduct accelerated stability studies (40°C/75% RH for 4 weeks) with periodic HPLC analysis to detect decomposition byproducts (e.g., nitro-group reduction or hydrolysis) .
Advanced Research Questions
Q. How to resolve contradictions in spectroscopic data during mechanistic studies of this compound’s reactivity?
- Answer : Adopt a mixed-methods approach:
- Experimental : Vary reaction conditions (solvent polarity, temperature) and track intermediates via in situ Raman spectroscopy.
- Computational : Use DFT to model transition states and compare with observed kinetics.
Triangulate findings with isotopic labeling (e.g., deuterated analogs ) to isolate pathways. Methodological rigor from qualitative research frameworks (e.g., iterative data collection/analysis ) applies here.
Q. What strategies address discrepancies in biological activity data across cell-line assays?
- Answer :
- Control for assay-specific variables : Cell permeability (logP adjustments), serum protein binding (ultrafiltration assays).
- Dose-response normalization : Use EC₅₀ values relative to internal standards (e.g., 4-(trifluoromethyl)phenylacetic acid derivatives ).
- Meta-analysis : Apply statistical models (e.g., mixed-effects regression) to reconcile inter-lab variability .
Q. How to design a study investigating the compound’s photostability under UV exposure?
- Answer :
- Experimental design : Use a solar simulator (ASTM G173 spectrum) with irradiance calibrated to 1.5 W/m². Monitor degradation via UV-Vis (λmax shifts) and LC-MS for photoproducts.
- Data interpretation : Compare quantum yield calculations with computational TD-DFT predictions of excited-state behavior .
- Replication : Include triplicate samples and negative controls (e.g., dark-stored analogs) .
Q. Data Contradiction Analysis
Q. Conflicting solubility profiles reported in polar vs. nonpolar solvents: How to determine the source of error?
- Answer :
- Method audit : Verify solvent purity (e.g., residual water in DMSO via Karl Fischer titration) and temperature control (±0.1°C).
- Alternative techniques : Compare shake-flask measurements with HPLC-derived solubility (standard curve method).
- Computational aid : Use COSMO-RS simulations to predict solubility parameters and identify outliers .
Q. Methodological Tables
Table 1. Cross-Validation of Purity Assessment Methods
Table 2. Stability Study Design Template
Condition | Duration | Analytical Endpoints | Acceptable Threshold |
---|---|---|---|
40°C/75% RH | 4 weeks | HPLC purity, mass balance | ≤5% degradation |
UV light (300–400 nm) | 48 hrs | UV-Vis spectral shift | Δλmax ≤5 nm |
Properties
CAS No. |
93105-66-9 |
---|---|
Molecular Formula |
C14H6N4O8 |
Molecular Weight |
358.22 g/mol |
IUPAC Name |
2,5,7-trinitro-9-oxofluorene-4-carboxamide |
InChI |
InChI=1S/C14H6N4O8/c15-14(20)9-3-5(16(21)22)1-7-11(9)12-8(13(7)19)2-6(17(23)24)4-10(12)18(25)26/h1-4H,(H2,15,20) |
InChI Key |
FXCNNLGCNUONLQ-UHFFFAOYSA-N |
Canonical SMILES |
C1=C(C=C(C2=C1C(=O)C3=C2C(=CC(=C3)[N+](=O)[O-])[N+](=O)[O-])C(=O)N)[N+](=O)[O-] |
Origin of Product |
United States |
Disclaimer and Information on In-Vitro Research Products
Please be aware that all articles and product information presented on BenchChem are intended solely for informational purposes. The products available for purchase on BenchChem are specifically designed for in-vitro studies, which are conducted outside of living organisms. In-vitro studies, derived from the Latin term "in glass," involve experiments performed in controlled laboratory settings using cells or tissues. It is important to note that these products are not categorized as medicines or drugs, and they have not received approval from the FDA for the prevention, treatment, or cure of any medical condition, ailment, or disease. We must emphasize that any form of bodily introduction of these products into humans or animals is strictly prohibited by law. It is essential to adhere to these guidelines to ensure compliance with legal and ethical standards in research and experimentation.