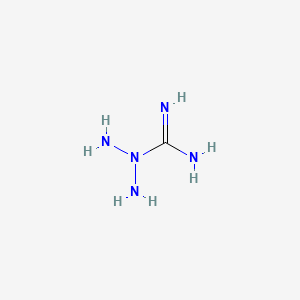
Diaminoguanidine
Overview
Description
Diaminoguanidine is an organic compound with the molecular formula CH₆N₄. It is a derivative of guanidine, characterized by the presence of two amino groups attached to the guanidine core. This compound is of significant interest due to its versatile applications in various fields, including chemistry, biology, and industry.
Preparation Methods
Synthetic Routes and Reaction Conditions: Diaminoguanidine can be synthesized through several methods. One common approach involves the reaction of cyanamide with hydrazine hydrate under acidic conditions to yield this compound. The reaction typically proceeds as follows: [ \text{NH}_2\text{CN} + \text{N}_2\text{H}_4 \cdot \text{H}_2\text{O} \rightarrow \text{NH}_2\text{C}(=NH)\text{NHNH}_2 ]
Industrial Production Methods: In industrial settings, this compound is often produced by the reaction of dicyandiamide with hydrazine hydrate. This method is favored due to its high yield and cost-effectiveness. The reaction conditions usually involve heating the reactants in an aqueous medium, followed by purification through recrystallization.
Chemical Reactions Analysis
Types of Reactions: Diaminoguanidine undergoes various chemical reactions, including:
Oxidation: this compound can be oxidized to form guanidine derivatives.
Reduction: It can be reduced to form hydrazine derivatives.
Substitution: this compound can participate in nucleophilic substitution reactions, where the amino groups are replaced by other functional groups.
Common Reagents and Conditions:
Oxidation: Common oxidizing agents include hydrogen peroxide and potassium permanganate.
Reduction: Reducing agents such as sodium borohydride and lithium aluminum hydride are often used.
Substitution: Reagents like alkyl halides and acyl chlorides are employed under basic conditions.
Major Products:
Oxidation: Guanidine derivatives.
Reduction: Hydrazine derivatives.
Substitution: Various substituted guanidine compounds.
Scientific Research Applications
Diaminoguanidine has a wide range of applications in scientific research:
Chemistry: It is used as a building block for the synthesis of complex organic molecules and as a ligand in coordination chemistry.
Biology: this compound derivatives are studied for their potential as enzyme inhibitors and antimicrobial agents.
Medicine: It has been investigated for its potential therapeutic effects, including its role as an inhibitor of nitric oxide synthase.
Industry: this compound-modified cellulose is used for the selective adsorption of heavy metals from aqueous solutions, making it valuable in environmental remediation.
Mechanism of Action
The mechanism of action of diaminoguanidine involves its interaction with various molecular targets. For instance, as an inhibitor of nitric oxide synthase, this compound binds to the enzyme’s active site, preventing the conversion of L-arginine to nitric oxide. This inhibition can modulate various physiological processes, including inflammation and vascular tone.
Comparison with Similar Compounds
Aminoguanidine: Known for its role as an inhibitor of advanced glycation end-products.
Nitroaminoguanidine: Studied for its potential as an explosive material.
Semicarbazide: Used in the synthesis of pharmaceuticals and as a reagent in analytical chemistry.
Diaminoguanidine’s unique structure and properties make it a valuable compound in various scientific and industrial applications.
Properties
CAS No. |
4364-78-7 |
---|---|
Molecular Formula |
CH7N5 |
Molecular Weight |
89.10 g/mol |
IUPAC Name |
1,2-diaminoguanidine |
InChI |
InChI=1S/CH7N5/c2-1(5-3)6-4/h3-4H2,(H3,2,5,6) |
InChI Key |
JGGFDEJXWLAQKR-UHFFFAOYSA-N |
Isomeric SMILES |
C(=N\N)(\N)/NN |
SMILES |
C(=N)(N)N(N)N |
Canonical SMILES |
C(=NN)(N)NN |
4364-78-7 | |
Related CAS |
36062-19-8 (mono-hydrochloride) 38360-74-6 (hydrochloride) |
Synonyms |
diaminoguanidine diaminoguanidine dihydrochloride diaminoguanidine hydrobromide diaminoguanidine hydrochloride diaminoguanidine monohydrochloride |
Origin of Product |
United States |
Retrosynthesis Analysis
AI-Powered Synthesis Planning: Our tool employs the Template_relevance Pistachio, Template_relevance Bkms_metabolic, Template_relevance Pistachio_ringbreaker, Template_relevance Reaxys, Template_relevance Reaxys_biocatalysis model, leveraging a vast database of chemical reactions to predict feasible synthetic routes.
One-Step Synthesis Focus: Specifically designed for one-step synthesis, it provides concise and direct routes for your target compounds, streamlining the synthesis process.
Accurate Predictions: Utilizing the extensive PISTACHIO, BKMS_METABOLIC, PISTACHIO_RINGBREAKER, REAXYS, REAXYS_BIOCATALYSIS database, our tool offers high-accuracy predictions, reflecting the latest in chemical research and data.
Strategy Settings
Precursor scoring | Relevance Heuristic |
---|---|
Min. plausibility | 0.01 |
Model | Template_relevance |
Template Set | Pistachio/Bkms_metabolic/Pistachio_ringbreaker/Reaxys/Reaxys_biocatalysis |
Top-N result to add to graph | 6 |
Feasible Synthetic Routes
Q1: What is the molecular formula and weight of Diaminoguanidine?
A1: this compound has a molecular formula of CH7N5 and a molecular weight of 91.11 g/mol.
Q2: What types of spectroscopic data are available for characterizing this compound?
A2: Researchers commonly employ techniques like Fourier-transform infrared spectroscopy (FTIR), nuclear magnetic resonance (NMR) spectroscopy, and mass spectrometry (MS) to characterize this compound. []
Q3: Is this compound more stable than guanidine and aminoguanidine?
A3: Yes, this compound exhibits greater stability in its protonated form compared to both guanidine and aminoguanidine. This enhanced stability is attributed to favorable intramolecular interactions present in protonated this compound. []
Q4: How do the electron delocalization properties of this compound compare to those of guanidine and aminoguanidine?
A4: Upon protonation, this compound demonstrates an increase in electron delocalization, similar to what is observed with guanidine and aminoguanidine. []
Q5: What are some potential applications of this compound?
A5: this compound derivatives show promise in several areas, including: * Animal feed additives: Studies suggest that this compound compounds can enhance production performance in livestock, such as ducks, pigs, and chickens. [] * Treatment of diabetes-associated complications: this compound has shown inhibitory effects on the formation of advanced glycation end products (AGEs), which are implicated in diabetic complications. [, ] * Antibacterial agents: Research indicates that this compound analogs can act as potent antibacterial agents, particularly against Gram-positive bacteria like methicillin-resistant Staphylococcus aureus (MRSA) and vancomycin-resistant Enterococcus (VRE). []
Q6: How does this compound interact with biological targets in the context of diabetes?
A6: this compound exhibits multiple mechanisms of action relevant to diabetes:
* **Inhibition of AGEs:** It effectively inhibits the formation of AGEs, which contribute to diabetic complications by damaging proteins and impairing their function. [, ] * **Aldose reductase inhibition:** It acts as a non-competitive inhibitor of aldose reductase, an enzyme involved in the polyol pathway of glucose metabolism. This pathway, when overactive in diabetes, contributes to the development of complications. []
Q7: Are there any known resistance mechanisms to this compound or its analogs in bacteria?
A7: While research on resistance mechanisms is ongoing, current studies haven't identified specific resistance mechanisms to this compound analogs in bacteria like MRSA. []
Q8: What is the role of this compound in the citrulline-nitric oxide cycle within pancreatic beta cells?
A8: this compound, alongside aminoguanidine and NG-amino-L-arginine, functions as a metabolism-based inactivator of nitric oxide synthase (NOS) isoforms. This inactivation process involves heme alteration in neuronal NOS (nNOS). [] Within pancreatic beta cells, this compound can inhibit the inducible form of NOS (iNOS), potentially mitigating beta-cell damage associated with Type 1 diabetes. []
Q9: How does this compound impact the gut microbiota in Type 2 diabetes?
A9: A study using a this compound-luteolin complex (DAGL·Cr) in a mouse model of Type 2 diabetes demonstrated its ability to favorably modulate the composition of the gut microbiota. DAGL·Cr treatment led to a reduction in the Firmicutes/Bacteroidetes ratio, an indicator often associated with improved metabolic health. Additionally, it increased the abundance of beneficial gut bacteria like Alistipes and Ruminiclostridium, potentially contributing to the observed improvements in blood sugar control. []
Q10: How do structural modifications of this compound affect its biological activity?
A10: Structure-activity relationship (SAR) studies have revealed that even subtle alterations to the this compound scaffold can significantly impact its activity:
- Alkylation of the imine carbon: This modification generally reduces anti-Giardia activity but allows for exploration of steric and electronic effects. []
- Substitution at the alpha position: While alpha-alkylation was detrimental to antidiabetic activity in analogs of 3-guanidinopropionic acid, regioisomeric aminoguanidinoacetic acid and diaminoguanidinoacetic acid analogs showed promising activity. []
Q11: Have computational chemistry methods been used in this compound research?
A11: Yes, computational techniques like Density Functional Theory (DFT) calculations have been employed to investigate the electronic structure and electron delocalization properties of this compound. [] Further studies utilize DFT to predict the properties of this compound-based energetic salts, highlighting its potential in developing high-energy materials. []
Q12: What analytical techniques are used to determine the presence and quantity of this compound?
A12: High-performance liquid chromatography (HPLC) is a commonly employed technique for quantifying this compound. It offers effective separation from other compounds like triaminoguanidine monohydrochloride. [] Additionally, nuclear magnetic resonance (NMR) spectroscopy proves valuable in simultaneously quantifying this compound alongside other nitrogen-containing compounds in complex matrices like milk. []
Q13: Have there been studies on the stability and formulation of this compound?
A13: While specific formulations are not extensively discussed in the provided research, its compatibility with various solvents and its ability to form stable salts with different counterions are evident. For instance, this compound readily forms salts with acids like hydrochloric acid and nitric acid. [, ] These salts exhibit varied properties and applications, indicating the potential for tailoring formulations to specific needs.
Q14: What are the SHE regulations surrounding the use of this compound?
A14: While specific SHE regulations are not detailed in the provided research, the potential toxicity of this compound and its derivatives, particularly in high concentrations, necessitates careful handling and adherence to relevant safety protocols. [, ]
Q15: What are some historical milestones in the research of this compound?
A16: Early research on this compound primarily focused on its synthesis and its application in forming diverse heterocyclic compounds, particularly triazoles and tetrazines. [, , , , , ] Over time, research expanded to explore its biological activities, revealing its potential in treating diabetes and as an antibacterial agent. [, , ]
Disclaimer and Information on In-Vitro Research Products
Please be aware that all articles and product information presented on BenchChem are intended solely for informational purposes. The products available for purchase on BenchChem are specifically designed for in-vitro studies, which are conducted outside of living organisms. In-vitro studies, derived from the Latin term "in glass," involve experiments performed in controlled laboratory settings using cells or tissues. It is important to note that these products are not categorized as medicines or drugs, and they have not received approval from the FDA for the prevention, treatment, or cure of any medical condition, ailment, or disease. We must emphasize that any form of bodily introduction of these products into humans or animals is strictly prohibited by law. It is essential to adhere to these guidelines to ensure compliance with legal and ethical standards in research and experimentation.