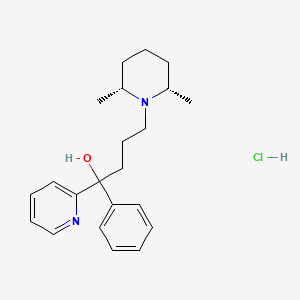
Pirmenol hydrochloride
Overview
Description
Pirmenol hydrochloride is an orally active antiarrhythmic agent . It was introduced in Japan in 1994 as a long-lasting Class Ia antiarrhythmic agent . The spectrum of antiarrhythmic activity of pirmenol includes atrial and ventricular arrhythmias of diverse etiology .
Synthesis Analysis
The synthesis of Pirmenol hydrochloride has been studied in humans to establish a minimum effective intravenous dose . Infusions of 70-150 mg were associated with a 90% or greater reduction in premature ventricular complexes (PVCs) nine of the twelve times they were administered to six patients .
Molecular Structure Analysis
The molecular formula of Pirmenol hydrochloride is C22H31ClN2O . Its molecular weight is 374.9 g/mol . The IUPAC name is 4-[(2S,6R)-2,6-dimethylpiperidin-1-yl]-1-phenyl-1-pyridin-2-ylbutan-1-ol;hydrochloride .
Chemical Reactions Analysis
Pirmenol hydrochloride inhibits IK.ACh (IC50: 0.1 μM) by blocking mAchR . This inhibition is a key part of its antiarrhythmic activity.
Physical And Chemical Properties Analysis
The physical and chemical properties of Pirmenol hydrochloride are as follows: Its molecular weight is 374.9 g/mol . It has a hydrogen bond donor count of 2 and a hydrogen bond acceptor count of 3 . It also has a rotatable bond count of 6 .
Scientific Research Applications
Cardiac Arrhythmias Treatment
Pirmenol hydrochloride: is primarily recognized for its role in treating various types of cardiac arrhythmias . It functions as a Class Ia antiarrhythmic agent , which means it can modify the electrical conduction within the heart to restore normal rhythm . Its efficacy has been demonstrated in both atrial and ventricular arrhythmias , and it is particularly useful due to its long half-life and low toxicity compared to other antiarrhythmic medications .
Electrophysiological Research
In electrophysiological studies, Pirmenol hydrochloride has been used to investigate the electrophysiological properties of cardiac cells . It has been shown to affect the fast sodium current and the delayed rectifying current in isolated rabbit Purkinje fibers, providing insights into its mechanism of action at the cellular level .
Pharmacokinetic Studies
Pirmenol hydrochloride has been the subject of pharmacokinetic studies to understand its absorption, distribution, metabolism, and excretion . These studies are crucial for determining the appropriate dosing regimens for therapeutic use and for understanding the drug’s safety profile .
Antiarrhythmic Efficacy
Clinical trials have evaluated the antiarrhythmic efficacy of Pirmenol hydrochloride, particularly in the treatment of premature ventricular contractions . These studies help in establishing the drug’s effectiveness in suppressing arrhythmias in human subjects .
Safety and Toxicity Assessments
Safety and toxicity studies are essential to ensure that Pirmenol hydrochloride can be used safely in humans. These studies assess the potential adverse effects on organs like the liver, heart, spleen, and kidneys, and evaluate the drug’s safety margin compared to other antiarrhythmic agents .
Mechanism of Action
Target of Action
Pirmenol hydrochloride primarily targets the muscarinic acetylcholine receptor M2 (mAchR) , potassium channels , and voltage-dependent calcium channels (VDCCs) . These targets play a crucial role in the electrophysiological properties of cardiac cells .
Mode of Action
Pirmenol hydrochloride inhibits the IK.ACh current by blocking the mAchR . It also exhibits potassium and sodium-channel blocking activity . In concentrations of 0.5–5 μmol/l, pirmenol causes a marked prolongation of the action potential duration in isolated rabbit Purkinje fibres .
Biochemical Pathways
The drug’s interaction with its targets affects the adrenergic signaling in cardiomyocytes and the dopaminergic synapse . Pirmenol hydrochloride causes a reduction of the steady-state sodium window current and/or of the slowly decaying components of the sodium current .
Pharmacokinetics
The steady-state pharmacokinetics of pirmenol was compared in healthy young (aged 18 to 45 y) and elderly subjects (over 65 y) given pirmenol HCl 100 mg every 12 h for a total of 14 doses . The results suggest that the dosage of pirmenol is unlikely to differ in young and elderly subjects .
Result of Action
Pirmenol hydrochloride’s action results in the suppression of arrhythmias. It is particularly effective against atrial fibrillation . The drug’s interaction with its targets leads to a decrease in the fast sodium current and a prolongation of the action potential duration .
properties
IUPAC Name |
4-[(2R,6S)-2,6-dimethylpiperidin-1-yl]-1-phenyl-1-pyridin-2-ylbutan-1-ol;hydrochloride | |
---|---|---|
Source | PubChem | |
URL | https://pubchem.ncbi.nlm.nih.gov | |
Description | Data deposited in or computed by PubChem | |
InChI |
InChI=1S/C22H30N2O.ClH/c1-18-10-8-11-19(2)24(18)17-9-15-22(25,20-12-4-3-5-13-20)21-14-6-7-16-23-21;/h3-7,12-14,16,18-19,25H,8-11,15,17H2,1-2H3;1H/t18-,19+,22?; | |
Source | PubChem | |
URL | https://pubchem.ncbi.nlm.nih.gov | |
Description | Data deposited in or computed by PubChem | |
InChI Key |
HFIHPVIVQSWZBV-ROSXHPEZSA-N | |
Source | PubChem | |
URL | https://pubchem.ncbi.nlm.nih.gov | |
Description | Data deposited in or computed by PubChem | |
Canonical SMILES |
CC1CCCC(N1CCCC(C2=CC=CC=C2)(C3=CC=CC=N3)O)C.Cl | |
Source | PubChem | |
URL | https://pubchem.ncbi.nlm.nih.gov | |
Description | Data deposited in or computed by PubChem | |
Isomeric SMILES |
C[C@@H]1CCC[C@@H](N1CCCC(C2=CC=CC=C2)(C3=CC=CC=N3)O)C.Cl | |
Source | PubChem | |
URL | https://pubchem.ncbi.nlm.nih.gov | |
Description | Data deposited in or computed by PubChem | |
Molecular Formula |
C22H31ClN2O | |
Source | PubChem | |
URL | https://pubchem.ncbi.nlm.nih.gov | |
Description | Data deposited in or computed by PubChem | |
DSSTOX Substance ID |
DTXSID6021169 | |
Record name | Pirmenol hydrochloride | |
Source | EPA DSSTox | |
URL | https://comptox.epa.gov/dashboard/DTXSID6021169 | |
Description | DSSTox provides a high quality public chemistry resource for supporting improved predictive toxicology. | |
Molecular Weight |
374.9 g/mol | |
Source | PubChem | |
URL | https://pubchem.ncbi.nlm.nih.gov | |
Description | Data deposited in or computed by PubChem | |
Product Name |
Pirmenol hydrochloride | |
CAS RN |
61477-94-9 | |
Record name | Pirmenol hydrochloride | |
Source | CAS Common Chemistry | |
URL | https://commonchemistry.cas.org/detail?cas_rn=61477-94-9 | |
Description | CAS Common Chemistry is an open community resource for accessing chemical information. Nearly 500,000 chemical substances from CAS REGISTRY cover areas of community interest, including common and frequently regulated chemicals, and those relevant to high school and undergraduate chemistry classes. This chemical information, curated by our expert scientists, is provided in alignment with our mission as a division of the American Chemical Society. | |
Explanation | The data from CAS Common Chemistry is provided under a CC-BY-NC 4.0 license, unless otherwise stated. | |
Record name | Pirmenol hydrochloride | |
Source | EPA DSSTox | |
URL | https://comptox.epa.gov/dashboard/DTXSID6021169 | |
Description | DSSTox provides a high quality public chemistry resource for supporting improved predictive toxicology. | |
Retrosynthesis Analysis
AI-Powered Synthesis Planning: Our tool employs the Template_relevance Pistachio, Template_relevance Bkms_metabolic, Template_relevance Pistachio_ringbreaker, Template_relevance Reaxys, Template_relevance Reaxys_biocatalysis model, leveraging a vast database of chemical reactions to predict feasible synthetic routes.
One-Step Synthesis Focus: Specifically designed for one-step synthesis, it provides concise and direct routes for your target compounds, streamlining the synthesis process.
Accurate Predictions: Utilizing the extensive PISTACHIO, BKMS_METABOLIC, PISTACHIO_RINGBREAKER, REAXYS, REAXYS_BIOCATALYSIS database, our tool offers high-accuracy predictions, reflecting the latest in chemical research and data.
Strategy Settings
Precursor scoring | Relevance Heuristic |
---|---|
Min. plausibility | 0.01 |
Model | Template_relevance |
Template Set | Pistachio/Bkms_metabolic/Pistachio_ringbreaker/Reaxys/Reaxys_biocatalysis |
Top-N result to add to graph | 6 |
Feasible Synthetic Routes
Disclaimer and Information on In-Vitro Research Products
Please be aware that all articles and product information presented on BenchChem are intended solely for informational purposes. The products available for purchase on BenchChem are specifically designed for in-vitro studies, which are conducted outside of living organisms. In-vitro studies, derived from the Latin term "in glass," involve experiments performed in controlled laboratory settings using cells or tissues. It is important to note that these products are not categorized as medicines or drugs, and they have not received approval from the FDA for the prevention, treatment, or cure of any medical condition, ailment, or disease. We must emphasize that any form of bodily introduction of these products into humans or animals is strictly prohibited by law. It is essential to adhere to these guidelines to ensure compliance with legal and ethical standards in research and experimentation.