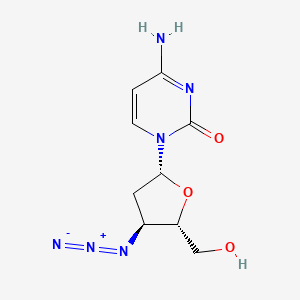
Cytidine, 3'-azido-2',3'-dideoxy-
Overview
Description
Cytidine, 3'-azido-2',3'-dideoxy-, also known as Cytidine, 3'-azido-2',3'-dideoxy-, is a useful research compound. Its molecular formula is C9H12N6O3 and its molecular weight is 252.23 g/mol. The purity is usually 95%.
BenchChem offers high-quality Cytidine, 3'-azido-2',3'-dideoxy- suitable for many research applications. Different packaging options are available to accommodate customers' requirements. Please inquire for more information about Cytidine, 3'-azido-2',3'-dideoxy- including the price, delivery time, and more detailed information at info@benchchem.com.
Scientific Research Applications
Antiretroviral Activity Against HIV
This compound, also known as AZT, shows potent antiretroviral activity against HIV in vitro and has been found to confer clinical benefits in patients with advanced acquired immune deficiency syndrome (AIDS) (Horwitz, 1989).
Synthesis and Antiviral Evaluation
Analogues of this compound have been synthesized and evaluated for potential anti-HIV activities. These analogues demonstrated significant anti-HIV-1 activity, indicating their potential as therapeutic agents (Lin et al., 1989).
Inhibitory Effects on HIV
Various 2',3'-dideoxynucleoside analogues, including those with 3'-azido modifications, have been synthesized with the aim of finding potent and selective inhibitors of HIV. Some of these analogues, like 3'-fluoro-2',3'-dideoxy-5-chloro-uridine, showed high selectivity and reduced toxicity, making them potential candidates for AIDS treatment (Van Aerschot, 1991).
Catabolic Disposition in Hepatocytes
The catabolic disposition of 3'-azido-2',3'-dideoxyuridine, a related compound, has been studied in hepatocytes, revealing insights into its metabolic pathways and potential implications for therapeutic use (Cretton et al., 1992).
Antineoplastic Activity
Some 3'-azido and 3'-amino nucleosides, including analogues of cytidine, have shown potent cytotoxic activity against certain cancer cells in vitro, suggesting their potential application in cancer chemotherapy (Lin & Mancini, 1983).
Synergistic Anti-HIV Effects
Studies have shown that certain combinations of nucleoside analogues, including those related to cytidine, can have synergistic effects against HIV, suggesting their potential for combination therapies (Malley et al., 1994).
Reduced Toxicity of Chlorinated Analogues
Chlorination of certain cytidine analogues has been found to reduce toxicity while maintaining anti-HIV activity, which is crucial for developing safer antiretroviral therapies (Van Aerschot et al., 1990).
Mechanism of Action
Target of Action
The primary target of 3’-Azido-ddC is the virus-encoded reverse transcriptase . This enzyme plays a crucial role in the replication of retroviruses such as the Human Immunodeficiency Virus (HIV), making it a key target for antiviral drugs .
Mode of Action
3’-Azido-ddC interacts with its target through a process known as phosphorylation . It is converted into its corresponding 5’-triphosphates, which act as inhibitors of the reverse transcriptase . This inhibitory action prevents the virus from replicating its genetic material, thereby halting the progression of the infection .
Biochemical Pathways
The action of 3’-Azido-ddC primarily affects the HIV replication pathway . By inhibiting the reverse transcriptase, it disrupts the conversion of viral RNA into DNA, a critical step in the replication of retroviruses . This results in a decrease in the production of new virus particles.
Result of Action
The molecular and cellular effects of 3’-Azido-ddC’s action primarily involve the inhibition of HIV replication . By blocking the action of reverse transcriptase, it prevents the virus from replicating its genetic material and producing new virus particles . This can lead to a reduction in viral load and a slowing of disease progression.
Future Directions
Cyclonucleosides like “Cytidine, 3’-azido-2’,3’-dideoxy-” have found wide applications in medicinal chemistry and biochemistry as biocides as well as enzyme inhibitors and molecular probes. They have also found use as convenient synthetic tools for the preparation of new nucleoside analogues, enabling structural modifications of both the sugar ring and heterocyclic base .
Biochemical Analysis
Cellular Effects
The effects of Cytidine, 3’-azido-2’,3’-dideoxy- on various cell types and cellular processes are profound. In human cells, this compound has been shown to inhibit telomerase activity, leading to telomere shortening and eventual cell senescence . Additionally, it affects cell signaling pathways and gene expression by incorporating into the DNA and RNA of cells, thereby disrupting normal cellular functions. This disruption can lead to altered cellular metabolism and reduced cell proliferation .
Molecular Mechanism
At the molecular level, Cytidine, 3’-azido-2’,3’-dideoxy- exerts its effects primarily through the inhibition of DNA synthesis. The incorporation of this compound into the growing DNA strand results in chain termination due to the absence of a 3’ hydroxyl group necessary for the formation of phosphodiester bonds. This mechanism is particularly effective against rapidly dividing cells, such as cancer cells and virus-infected cells . Additionally, Cytidine, 3’-azido-2’,3’-dideoxy- selectively inhibits telomerase, an enzyme responsible for maintaining telomere length, thereby inducing cellular senescence .
Temporal Effects in Laboratory Settings
In laboratory settings, the effects of Cytidine, 3’-azido-2’,3’-dideoxy- have been observed to change over time. The compound is relatively stable under standard storage conditions but can degrade when exposed to light and high temperatures . Long-term studies have shown that continuous exposure to this compound can lead to cumulative effects on cellular function, including persistent inhibition of DNA synthesis and telomerase activity . These effects are crucial for understanding the long-term implications of using this compound in therapeutic applications.
Dosage Effects in Animal Models
The effects of Cytidine, 3’-azido-2’,3’-dideoxy- vary with different dosages in animal models. At lower doses, the compound effectively inhibits viral replication and reduces tumor growth without significant toxicity . At higher doses, it can cause adverse effects, including bone marrow suppression and hepatotoxicity . These findings highlight the importance of optimizing dosage to balance efficacy and safety in therapeutic applications.
Metabolic Pathways
Cytidine, 3’-azido-2’,3’-dideoxy- is involved in several metabolic pathways, primarily those related to nucleotide metabolism. The compound is phosphorylated by cellular kinases to form its active triphosphate form, which is then incorporated into DNA and RNA . This incorporation disrupts normal nucleotide metabolism and can lead to the accumulation of abnormal nucleotides, affecting overall metabolic flux .
Transport and Distribution
Within cells and tissues, Cytidine, 3’-azido-2’,3’-dideoxy- is transported and distributed through various mechanisms. It is taken up by cells via nucleoside transporters and is distributed to different cellular compartments . The compound’s distribution is influenced by its interactions with binding proteins and its ability to cross cellular membranes . Understanding these transport and distribution mechanisms is essential for optimizing its therapeutic use.
Subcellular Localization
The subcellular localization of Cytidine, 3’-azido-2’,3’-dideoxy- is critical for its activity and function. The compound is primarily localized in the nucleus, where it exerts its effects on DNA synthesis and telomerase activity . Additionally, it can be found in the cytoplasm, where it may interact with RNA and other cellular components . The localization is influenced by targeting signals and post-translational modifications that direct the compound to specific cellular compartments .
Properties
IUPAC Name |
4-amino-1-[4-azido-5-(hydroxymethyl)oxolan-2-yl]pyrimidin-2-one | |
---|---|---|
Details | Computed by Lexichem TK 2.7.0 (PubChem release 2021.05.07) | |
Source | PubChem | |
URL | https://pubchem.ncbi.nlm.nih.gov | |
Description | Data deposited in or computed by PubChem | |
InChI |
InChI=1S/C9H12N6O3/c10-7-1-2-15(9(17)12-7)8-3-5(13-14-11)6(4-16)18-8/h1-2,5-6,8,16H,3-4H2,(H2,10,12,17) | |
Details | Computed by InChI 1.0.6 (PubChem release 2021.05.07) | |
Source | PubChem | |
URL | https://pubchem.ncbi.nlm.nih.gov | |
Description | Data deposited in or computed by PubChem | |
InChI Key |
YIEFKLOVIROQIL-UHFFFAOYSA-N | |
Details | Computed by InChI 1.0.6 (PubChem release 2021.05.07) | |
Source | PubChem | |
URL | https://pubchem.ncbi.nlm.nih.gov | |
Description | Data deposited in or computed by PubChem | |
Canonical SMILES |
C1C(C(OC1N2C=CC(=NC2=O)N)CO)N=[N+]=[N-] | |
Details | Computed by OEChem 2.3.0 (PubChem release 2021.05.07) | |
Source | PubChem | |
URL | https://pubchem.ncbi.nlm.nih.gov | |
Description | Data deposited in or computed by PubChem | |
Molecular Formula |
C9H12N6O3 | |
Details | Computed by PubChem 2.1 (PubChem release 2021.05.07) | |
Source | PubChem | |
URL | https://pubchem.ncbi.nlm.nih.gov | |
Description | Data deposited in or computed by PubChem | |
Molecular Weight |
252.23 g/mol | |
Details | Computed by PubChem 2.1 (PubChem release 2021.05.07) | |
Source | PubChem | |
URL | https://pubchem.ncbi.nlm.nih.gov | |
Description | Data deposited in or computed by PubChem | |
CAS No. |
84472-89-9 | |
Record name | NSC382318 | |
Source | DTP/NCI | |
URL | https://dtp.cancer.gov/dtpstandard/servlet/dwindex?searchtype=NSC&outputformat=html&searchlist=382318 | |
Description | The NCI Development Therapeutics Program (DTP) provides services and resources to the academic and private-sector research communities worldwide to facilitate the discovery and development of new cancer therapeutic agents. | |
Explanation | Unless otherwise indicated, all text within NCI products is free of copyright and may be reused without our permission. Credit the National Cancer Institute as the source. | |
Retrosynthesis Analysis
AI-Powered Synthesis Planning: Our tool employs the Template_relevance Pistachio, Template_relevance Bkms_metabolic, Template_relevance Pistachio_ringbreaker, Template_relevance Reaxys, Template_relevance Reaxys_biocatalysis model, leveraging a vast database of chemical reactions to predict feasible synthetic routes.
One-Step Synthesis Focus: Specifically designed for one-step synthesis, it provides concise and direct routes for your target compounds, streamlining the synthesis process.
Accurate Predictions: Utilizing the extensive PISTACHIO, BKMS_METABOLIC, PISTACHIO_RINGBREAKER, REAXYS, REAXYS_BIOCATALYSIS database, our tool offers high-accuracy predictions, reflecting the latest in chemical research and data.
Strategy Settings
Precursor scoring | Relevance Heuristic |
---|---|
Min. plausibility | 0.01 |
Model | Template_relevance |
Template Set | Pistachio/Bkms_metabolic/Pistachio_ringbreaker/Reaxys/Reaxys_biocatalysis |
Top-N result to add to graph | 6 |
Feasible Synthetic Routes
Q1: How does 3′-azido-ddC interact with HIV-1 reverse transcriptase, and what are the downstream effects?
A1: 3′-azido-ddC, after being phosphorylated to its active triphosphate form inside the cell, inhibits HIV-1 reverse transcriptase (RT), the viral enzyme essential for converting viral RNA into DNA. It does so by competing with the natural nucleotide, deoxycytidine triphosphate (dCTP), for incorporation into the growing viral DNA chain. Due to the presence of an azido group (N3) instead of a hydroxyl group (OH) at the 3' position of the sugar moiety, 3′-azido-ddC acts as a chain terminator, halting further DNA synthesis. [, ] This effectively blocks viral replication.
Q2: What specific mutations in HIV-1 RT are associated with resistance to 3′-azido-ddC?
A2: Research indicates that the V75I mutation in HIV-1 RT confers resistance to 3′-azido-ddC. This mutation was observed in in vitro selection experiments where HIV-1 was exposed to increasing concentrations of 3′-azido-ddC. Viruses carrying the V75I mutation showed a 5.9-fold increase in resistance to 3′-azido-ddC compared to the wild-type virus. []
Q3: How does the nucleoside base influence the development of resistance to 3′-azido-2′,3′-dideoxynucleosides?
A3: Studies using different 3′-azido-2′,3′-dideoxynucleosides, each with a different nucleobase (guanosine, cytidine, adenosine), have shown that the base significantly impacts the resistance profile. For instance, while a specific mutation (V75I) confers resistance to 3′-azido-ddC, different mutations emerge when the virus is challenged with 3′-azido-ddG (3′-azido-2′,3′-dideoxyguanosine). Interestingly, researchers were unable to induce resistance to 3′-azido-ddA (3′-azido-2′,3′-dideoxyadenosine), even at high intracellular concentrations of its active triphosphate form. [, ] This highlights the importance of the nucleobase in both the drug's mechanism of action and the development of resistance. This information can be valuable in designing new nucleoside RT inhibitors.
Disclaimer and Information on In-Vitro Research Products
Please be aware that all articles and product information presented on BenchChem are intended solely for informational purposes. The products available for purchase on BenchChem are specifically designed for in-vitro studies, which are conducted outside of living organisms. In-vitro studies, derived from the Latin term "in glass," involve experiments performed in controlled laboratory settings using cells or tissues. It is important to note that these products are not categorized as medicines or drugs, and they have not received approval from the FDA for the prevention, treatment, or cure of any medical condition, ailment, or disease. We must emphasize that any form of bodily introduction of these products into humans or animals is strictly prohibited by law. It is essential to adhere to these guidelines to ensure compliance with legal and ethical standards in research and experimentation.