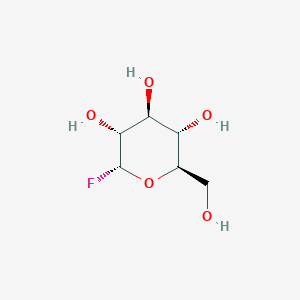
alpha-D-Glucopyranosyl fluoride
Overview
Description
Synthesis Analysis
Alpha-D-Glucopyranosyl fluoride can be synthesized through a stepwise solvent-promoted SNi reaction, displaying mechanistic implications for retaining glycosyltransferases. The solvolysis of this compound in hexafluoro-2-propanol yields two products, highlighting the importance of solvent effects in the synthesis and rearrangement of glycosyl fluorides (Chan, Tang, & Bennet, 2012).
Molecular Structure Analysis
The molecular structure of this compound has been elucidated through various spectroscopic and crystallographic techniques, revealing its conformation and stereochemistry. X-ray analyses of peracetylated this compound demonstrate the glycopyranosyl ring's regular (4)C1 chair conformation, with the anomeric fluoride adopting an axial orientation, highlighting the strong anomeric effect influencing the molecule's structure (Dedola, Hughes, & Field, 2010).
Chemical Reactions and Properties
This compound acts as a glucopyranosyl donor in various enzymatic reactions, such as those catalyzed by sucrose phosphorylase, indicating its versatility as a substrate for enzyme-mediated glycosylation processes. The enzyme catalyzes the hydrolysis of glucosyl fluoride at rates comparable to sucrose, underscoring its utility as a glucose donor in biochemical transformations (Gold & Osber, 1971).
Scientific Research Applications
Fluorine-19 NMR Studies : Alpha-D-Glucopyranosyl fluoride's transport in human erythrocytes was studied using fluorine-19 magnetization transfer, although its exchange rate was too slow for effective measurement. This study highlighted significant differences in the permeabilities of alpha and beta forms of glucopyranosyl fluorides, which could be explained by an alternating conformation model involving selective binding preferences based on the conformation and site of fluorination (London & Gabel, 1995).
Enzyme Inhibition Studies : this compound strongly inhibits alpha-glucan phosphorylase from rabbit muscle and, to a lesser extent, from potato tubers. The study explored its competitive inhibition characteristics, providing insights into the binding interactions with the enzyme (Ariki & Fukui, 1975).
Glycosyltransferase Research : This compound served as a D-glucopyranosyl donor for the glycosyltransferase from Streptococcus mutans, resulting in a D-glucan of high molecular weight and fluoride ion. The study also explored the inhibitory effects of various monosaccharides on this reaction (Figures & Edwards, 1976).
Sucrose Phosphorylase Substrate : this compound was identified as a substrate for sucrose phosphorylase from Pseudomonas saccharophila, displaying comparable kinetic properties to sucrose (Gold & Osber, 1971).
Mechanism of Action
Target of Action
Alpha-D-Glucopyranosyl fluoride primarily targets glycoside hydrolases (GHs) . These enzymes are found in all biological kingdoms and play a crucial role in carbohydrate metabolism . They catalyze the biochemical transfer of carbohydrate units from donor sugars to acceptor biomolecules or water .
Mode of Action
This compound interacts with its targets, the glycoside hydrolases, through a process of hydrolysis . This interaction involves the departure of a fluoride ion within a glycoside hydrolase active site that stabilizes pyranosylium ion-like transition states . The catalysis is driven solely by H-bonding assistance from an enzymatic carboxylic acid residue . No assistance is required from the bound nucleophile, which in these two cases is either a water molecule (GH15) or a sugar hydroxyl group (GH55) .
Biochemical Pathways
The hydrolysis of this compound by glycoside hydrolases affects the carbohydrate metabolism pathway . This process results in the biochemical transfer of carbohydrate units from donor sugars to acceptor biomolecules or water . The specific downstream effects of this process depend on the specific glycoside hydrolase involved and the broader metabolic context.
Pharmacokinetics
The compound’s interaction with glycoside hydrolases suggests that it may be metabolized through the carbohydrate metabolism pathway .
Result of Action
The hydrolysis of this compound by glycoside hydrolases results in the formation of D-glucose . This process involves either configurational inversion or retention, depending on the specific enzyme involved . The transition states for both enzymatic reactions are essentially identical .
Future Directions
In addition to alpha-D-fluoroglucose, the glycosyl donors alpha-D-fluoromaltose and alpha-D-fluorogalactose underwent glycosylation with Boc-Tyr-OH under the reaction conditions, providing O-glycosyl . This suggests potential future directions for research and applications of alpha-D-Glucopyranosyl fluoride and similar compounds.
properties
IUPAC Name |
(2R,3R,4S,5S,6R)-2-fluoro-6-(hydroxymethyl)oxane-3,4,5-triol | |
---|---|---|
Source | PubChem | |
URL | https://pubchem.ncbi.nlm.nih.gov | |
Description | Data deposited in or computed by PubChem | |
InChI |
InChI=1S/C6H11FO5/c7-6-5(11)4(10)3(9)2(1-8)12-6/h2-6,8-11H,1H2/t2-,3-,4+,5-,6+/m1/s1 | |
Source | PubChem | |
URL | https://pubchem.ncbi.nlm.nih.gov | |
Description | Data deposited in or computed by PubChem | |
InChI Key |
ATMYEINZLWEOQU-DVKNGEFBSA-N | |
Source | PubChem | |
URL | https://pubchem.ncbi.nlm.nih.gov | |
Description | Data deposited in or computed by PubChem | |
Canonical SMILES |
C(C1C(C(C(C(O1)F)O)O)O)O | |
Source | PubChem | |
URL | https://pubchem.ncbi.nlm.nih.gov | |
Description | Data deposited in or computed by PubChem | |
Isomeric SMILES |
C([C@@H]1[C@H]([C@@H]([C@H]([C@H](O1)F)O)O)O)O | |
Source | PubChem | |
URL | https://pubchem.ncbi.nlm.nih.gov | |
Description | Data deposited in or computed by PubChem | |
Molecular Formula |
C6H11FO5 | |
Source | PubChem | |
URL | https://pubchem.ncbi.nlm.nih.gov | |
Description | Data deposited in or computed by PubChem | |
Molecular Weight |
182.15 g/mol | |
Source | PubChem | |
URL | https://pubchem.ncbi.nlm.nih.gov | |
Description | Data deposited in or computed by PubChem | |
CAS RN |
2106-10-7 | |
Record name | 1-Fluoro-1-deoxy-?-D-glucose | |
Source | European Chemicals Agency (ECHA) | |
URL | https://echa.europa.eu/information-on-chemicals | |
Description | The European Chemicals Agency (ECHA) is an agency of the European Union which is the driving force among regulatory authorities in implementing the EU's groundbreaking chemicals legislation for the benefit of human health and the environment as well as for innovation and competitiveness. | |
Explanation | Use of the information, documents and data from the ECHA website is subject to the terms and conditions of this Legal Notice, and subject to other binding limitations provided for under applicable law, the information, documents and data made available on the ECHA website may be reproduced, distributed and/or used, totally or in part, for non-commercial purposes provided that ECHA is acknowledged as the source: "Source: European Chemicals Agency, http://echa.europa.eu/". Such acknowledgement must be included in each copy of the material. ECHA permits and encourages organisations and individuals to create links to the ECHA website under the following cumulative conditions: Links can only be made to webpages that provide a link to the Legal Notice page. | |
Retrosynthesis Analysis
AI-Powered Synthesis Planning: Our tool employs the Template_relevance Pistachio, Template_relevance Bkms_metabolic, Template_relevance Pistachio_ringbreaker, Template_relevance Reaxys, Template_relevance Reaxys_biocatalysis model, leveraging a vast database of chemical reactions to predict feasible synthetic routes.
One-Step Synthesis Focus: Specifically designed for one-step synthesis, it provides concise and direct routes for your target compounds, streamlining the synthesis process.
Accurate Predictions: Utilizing the extensive PISTACHIO, BKMS_METABOLIC, PISTACHIO_RINGBREAKER, REAXYS, REAXYS_BIOCATALYSIS database, our tool offers high-accuracy predictions, reflecting the latest in chemical research and data.
Strategy Settings
Precursor scoring | Relevance Heuristic |
---|---|
Min. plausibility | 0.01 |
Model | Template_relevance |
Template Set | Pistachio/Bkms_metabolic/Pistachio_ringbreaker/Reaxys/Reaxys_biocatalysis |
Top-N result to add to graph | 6 |
Feasible Synthetic Routes
Disclaimer and Information on In-Vitro Research Products
Please be aware that all articles and product information presented on BenchChem are intended solely for informational purposes. The products available for purchase on BenchChem are specifically designed for in-vitro studies, which are conducted outside of living organisms. In-vitro studies, derived from the Latin term "in glass," involve experiments performed in controlled laboratory settings using cells or tissues. It is important to note that these products are not categorized as medicines or drugs, and they have not received approval from the FDA for the prevention, treatment, or cure of any medical condition, ailment, or disease. We must emphasize that any form of bodily introduction of these products into humans or animals is strictly prohibited by law. It is essential to adhere to these guidelines to ensure compliance with legal and ethical standards in research and experimentation.