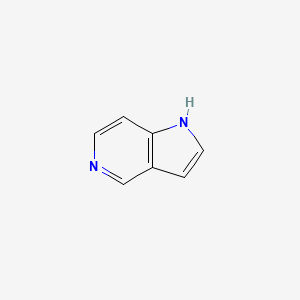
5-Azaindole
Overview
Description
5-Azaindole, also known as pyrrolo[3,2-b]pyridine, is a heterocyclic compound that consists of a pyridine ring fused to a pyrrole ring. This compound is a positional isomer of indole, where the nitrogen atom is located at the 5-position of the pyridine ring. This compound and its derivatives are known for their significant biological activities and have been extensively studied for their potential therapeutic applications .
Mechanism of Action
Target of Action
5-Azaindole, a structural isomer of azaindole, is known to be a key pharmacophore in many bioactive compounds . It has been recognized as a privileged structure in biological process modulation, particularly in the field of kinases . Kinases are the primary targets of this compound, and they play a crucial role in various biological processes, including cell growth, differentiation, metabolism, and apoptosis .
Mode of Action
This compound interacts with its targets, primarily kinases, by binding to their ATP active site . This interaction results in the inhibition of kinase activity, thereby modulating the biological processes controlled by these kinases . The exact mode of action can vary depending on the specific kinase target and the structural modifications of the this compound molecule.
Biochemical Pathways
The biochemical pathways affected by this compound are primarily those regulated by kinases. For instance, the RAF/MEK/ERK pathway, which is crucial for cell proliferation and survival, can be modulated by this compound-based kinase inhibitors . By inhibiting kinase activity, this compound can disrupt these pathways, leading to downstream effects such as the suppression of cell growth and induction of apoptosis .
Pharmacokinetics
The pharmacokinetics of this compound, including its absorption, distribution, metabolism, and excretion (ADME) properties, can significantly impact its bioavailability and therapeutic efficacy . . It’s worth noting that the azaindole core can be used to modulate and finely tune these properties, making it a valuable scaffold in drug design .
Result of Action
The molecular and cellular effects of this compound’s action primarily involve the modulation of kinase activity. By inhibiting kinases, this compound can suppress cell proliferation, induce apoptosis, and potentially exert anti-cancer effects . These effects can vary depending on the specific kinase target and the cellular context.
Action Environment
The action, efficacy, and stability of this compound can be influenced by various environmental factors. For instance, the photodynamics of azaindoles, including this compound, have been shown to be influenced by the polarity of the environment . Moreover, the presence of protic media (methanol) can trigger reactive channels (proton transfer) upon electronic excitation, leading to different photodynamical behavior .
Biochemical Analysis
Biochemical Properties
5-Azaindole plays a crucial role in various biochemical reactions, particularly as a kinase inhibitor. It interacts with several enzymes, proteins, and other biomolecules, influencing their activity and function. One of the primary interactions of this compound is with protein kinases, where it binds to the ATP active site, forming hydrogen bonds through the nitrogen of the pyridine and the secondary amine linked to the azaindole scaffold . This interaction inhibits the kinase activity, thereby modulating various cellular signaling pathways. Additionally, this compound has been shown to inhibit factor VIIa, an enzyme involved in the coagulation cascade .
Cellular Effects
This compound exerts significant effects on various types of cells and cellular processes. It influences cell function by modulating cell signaling pathways, gene expression, and cellular metabolism. For instance, this compound has been found to regulate apoptosis, cell cycle progression, cytoskeletal rearrangement, differentiation, development, immune response, nervous system function, and transcription . By inhibiting specific kinases, this compound can alter the phosphorylation status of key signaling molecules, leading to changes in gene expression and cellular behavior.
Molecular Mechanism
The molecular mechanism of this compound involves its binding interactions with biomolecules, enzyme inhibition, and changes in gene expression. At the molecular level, this compound binds to the ATP active site of protein kinases, forming hydrogen bonds that inhibit kinase activity . This inhibition prevents the phosphorylation of downstream signaling molecules, thereby modulating various cellular processes. Additionally, this compound can influence gene expression by altering the activity of transcription factors and other regulatory proteins.
Temporal Effects in Laboratory Settings
In laboratory settings, the effects of this compound can change over time due to its stability, degradation, and long-term impact on cellular function. Studies have shown that this compound is relatively stable under standard laboratory conditions, but its activity may decrease over extended periods due to degradation . Long-term exposure to this compound can lead to sustained inhibition of kinase activity, resulting in prolonged changes in cellular signaling and function.
Dosage Effects in Animal Models
The effects of this compound vary with different dosages in animal models. At lower doses, this compound can effectively inhibit kinase activity without causing significant toxicity . At higher doses, it may exhibit toxic or adverse effects, such as hepatotoxicity and nephrotoxicity . The therapeutic window of this compound is therefore critical in determining its efficacy and safety in preclinical studies.
Metabolic Pathways
This compound is involved in several metabolic pathways, interacting with various enzymes and cofactors. It is primarily metabolized by cytochrome P450 enzymes in the liver, leading to the formation of several metabolites . These metabolites can further influence metabolic flux and metabolite levels, impacting overall cellular metabolism. The interaction of this compound with metabolic enzymes can also affect its pharmacokinetics and bioavailability.
Transport and Distribution
Within cells and tissues, this compound is transported and distributed through various mechanisms. It interacts with specific transporters and binding proteins that facilitate its uptake and localization . The distribution of this compound within different cellular compartments can influence its activity and function. For example, its accumulation in the nucleus can enhance its effects on gene expression and transcriptional regulation.
Subcellular Localization
The subcellular localization of this compound is critical for its activity and function. It can be directed to specific compartments or organelles through targeting signals and post-translational modifications . For instance, the presence of nuclear localization signals can facilitate the transport of this compound to the nucleus, where it can interact with transcription factors and other nuclear proteins. This localization is essential for its role in regulating gene expression and cellular signaling.
Preparation Methods
Synthetic Routes and Reaction Conditions
The synthesis of 5-Azaindole can be achieved through various methods. One common approach involves the cyclization of 3-methyl-4-nitropyridine with N,N-dialkylformamide dialkyl acetal, followed by reduction and cyclization using a palladium-containing catalyst and hydrogen donor . Another method involves a site-selective palladium-catalyzed carbon-nitrogen coupling reaction of 3,4-dibromopyridine with 4-toluidine, followed by carbon-carbon coupling and cyclization with phenylacetylene .
Industrial Production Methods
Industrial production of this compound typically involves large-scale synthesis using optimized reaction conditions to ensure high yield and purity. For example, the preparation method developed by Zhejiang University involves the reaction of 3-methyl-4-nitropyridine with N,N-dialkylformamide dioxane acetal in a non-proton polar solvent at 100-150°C, followed by reduction and cyclization using Raney nickel and hydrogen donor .
Chemical Reactions Analysis
Types of Reactions
5-Azaindole undergoes various chemical reactions, including:
Oxidation: Oxidation of this compound can be achieved using reagents such as hydrogen peroxide.
Reduction: Reduction reactions often involve the use of palladium-containing catalysts and hydrogen donors.
Substitution: Nucleophilic substitution reactions can occur at the halogenated positions of this compound derivatives.
Common Reagents and Conditions
Oxidation: Hydrogen peroxide is commonly used as an oxidizing agent.
Reduction: Palladium-containing catalysts and hydrogen donors are used for reduction reactions.
Substitution: Alkoxy groups and various amines are used for nucleophilic substitution reactions.
Major Products Formed
The major products formed from these reactions include various substituted azaindole derivatives, which can exhibit different biological activities depending on the nature of the substituents.
Scientific Research Applications
5-Azaindole and its derivatives have a wide range of scientific research applications:
Chemistry: this compound is used as a building block in the synthesis of various heterocyclic compounds.
Biology: It is used in the study of biological processes and as a probe for investigating enzyme activities.
Comparison with Similar Compounds
Similar Compounds
- 4-Azaindole
- 6-Azaindole
- 7-Azaindole
Comparison
5-Azaindole is unique due to the position of the nitrogen atom at the 5-position of the pyridine ring. This positional isomerism can influence its chemical reactivity and biological activity. Compared to other azaindoles, this compound exhibits distinct binding affinities and selectivities towards specific molecular targets, making it a valuable scaffold in drug discovery .
Properties
IUPAC Name |
1H-pyrrolo[3,2-c]pyridine | |
---|---|---|
Source | PubChem | |
URL | https://pubchem.ncbi.nlm.nih.gov | |
Description | Data deposited in or computed by PubChem | |
InChI |
InChI=1S/C7H6N2/c1-4-9-7-2-3-8-5-6(1)7/h1-5,9H | |
Source | PubChem | |
URL | https://pubchem.ncbi.nlm.nih.gov | |
Description | Data deposited in or computed by PubChem | |
InChI Key |
SRSKXJVMVSSSHB-UHFFFAOYSA-N | |
Source | PubChem | |
URL | https://pubchem.ncbi.nlm.nih.gov | |
Description | Data deposited in or computed by PubChem | |
Canonical SMILES |
C1=CNC2=C1C=NC=C2 | |
Source | PubChem | |
URL | https://pubchem.ncbi.nlm.nih.gov | |
Description | Data deposited in or computed by PubChem | |
Molecular Formula |
C7H6N2 | |
Source | PubChem | |
URL | https://pubchem.ncbi.nlm.nih.gov | |
Description | Data deposited in or computed by PubChem | |
DSSTOX Substance ID |
DTXSID80181563 | |
Record name | 1H-Pyrrolo(3,2-c)pyridine | |
Source | EPA DSSTox | |
URL | https://comptox.epa.gov/dashboard/DTXSID80181563 | |
Description | DSSTox provides a high quality public chemistry resource for supporting improved predictive toxicology. | |
Molecular Weight |
118.14 g/mol | |
Source | PubChem | |
URL | https://pubchem.ncbi.nlm.nih.gov | |
Description | Data deposited in or computed by PubChem | |
CAS No. |
271-34-1 | |
Record name | 1H-Pyrrolo[3,2-c]pyridine | |
Source | CAS Common Chemistry | |
URL | https://commonchemistry.cas.org/detail?cas_rn=271-34-1 | |
Description | CAS Common Chemistry is an open community resource for accessing chemical information. Nearly 500,000 chemical substances from CAS REGISTRY cover areas of community interest, including common and frequently regulated chemicals, and those relevant to high school and undergraduate chemistry classes. This chemical information, curated by our expert scientists, is provided in alignment with our mission as a division of the American Chemical Society. | |
Explanation | The data from CAS Common Chemistry is provided under a CC-BY-NC 4.0 license, unless otherwise stated. | |
Record name | 1H-Pyrrolo(3,2-c)pyridine | |
Source | ChemIDplus | |
URL | https://pubchem.ncbi.nlm.nih.gov/substance/?source=chemidplus&sourceid=0000271341 | |
Description | ChemIDplus is a free, web search system that provides access to the structure and nomenclature authority files used for the identification of chemical substances cited in National Library of Medicine (NLM) databases, including the TOXNET system. | |
Record name | 1H-Pyrrolo(3,2-c)pyridine | |
Source | EPA DSSTox | |
URL | https://comptox.epa.gov/dashboard/DTXSID80181563 | |
Description | DSSTox provides a high quality public chemistry resource for supporting improved predictive toxicology. | |
Record name | 1H-Pyrrolo[3,2-c]pyridine | |
Source | European Chemicals Agency (ECHA) | |
URL | https://echa.europa.eu/information-on-chemicals | |
Description | The European Chemicals Agency (ECHA) is an agency of the European Union which is the driving force among regulatory authorities in implementing the EU's groundbreaking chemicals legislation for the benefit of human health and the environment as well as for innovation and competitiveness. | |
Explanation | Use of the information, documents and data from the ECHA website is subject to the terms and conditions of this Legal Notice, and subject to other binding limitations provided for under applicable law, the information, documents and data made available on the ECHA website may be reproduced, distributed and/or used, totally or in part, for non-commercial purposes provided that ECHA is acknowledged as the source: "Source: European Chemicals Agency, http://echa.europa.eu/". Such acknowledgement must be included in each copy of the material. ECHA permits and encourages organisations and individuals to create links to the ECHA website under the following cumulative conditions: Links can only be made to webpages that provide a link to the Legal Notice page. | |
Synthesis routes and methods I
Procedure details
Synthesis routes and methods II
Procedure details
Synthesis routes and methods III
Procedure details
Synthesis routes and methods IV
Procedure details
Retrosynthesis Analysis
AI-Powered Synthesis Planning: Our tool employs the Template_relevance Pistachio, Template_relevance Bkms_metabolic, Template_relevance Pistachio_ringbreaker, Template_relevance Reaxys, Template_relevance Reaxys_biocatalysis model, leveraging a vast database of chemical reactions to predict feasible synthetic routes.
One-Step Synthesis Focus: Specifically designed for one-step synthesis, it provides concise and direct routes for your target compounds, streamlining the synthesis process.
Accurate Predictions: Utilizing the extensive PISTACHIO, BKMS_METABOLIC, PISTACHIO_RINGBREAKER, REAXYS, REAXYS_BIOCATALYSIS database, our tool offers high-accuracy predictions, reflecting the latest in chemical research and data.
Strategy Settings
Precursor scoring | Relevance Heuristic |
---|---|
Min. plausibility | 0.01 |
Model | Template_relevance |
Template Set | Pistachio/Bkms_metabolic/Pistachio_ringbreaker/Reaxys/Reaxys_biocatalysis |
Top-N result to add to graph | 6 |
Feasible Synthetic Routes
Disclaimer and Information on In-Vitro Research Products
Please be aware that all articles and product information presented on BenchChem are intended solely for informational purposes. The products available for purchase on BenchChem are specifically designed for in-vitro studies, which are conducted outside of living organisms. In-vitro studies, derived from the Latin term "in glass," involve experiments performed in controlled laboratory settings using cells or tissues. It is important to note that these products are not categorized as medicines or drugs, and they have not received approval from the FDA for the prevention, treatment, or cure of any medical condition, ailment, or disease. We must emphasize that any form of bodily introduction of these products into humans or animals is strictly prohibited by law. It is essential to adhere to these guidelines to ensure compliance with legal and ethical standards in research and experimentation.