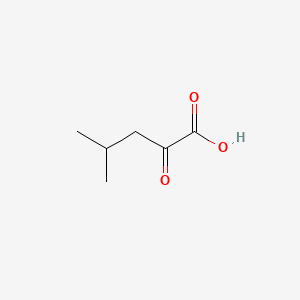
4-Methyl-2-oxopentanoic acid
Overview
Description
Mechanism of Action
Target of Action
4-Methyl-2-oxopentanoic acid, also known as α-Ketoisocaproic acid, is a metabolic intermediate in the metabolic pathway for L-leucine . Leucine is an essential amino acid, and its degradation is critical for many biological duties .
Mode of Action
It is known to be involved in the catabolism of branched-chain amino acids (bcaas) such as leucine .
Biochemical Pathways
This compound is a key player in the metabolic pathway of the essential amino acid leucine . It is produced during the catabolism of leucine and is further metabolized by various enzymes in the body . It has been found to accumulate in certain metabolic disorders such as maple syrup urine disease .
Pharmacokinetics
It is known to be a metabolite produced during metabolic reactions in humans .
Result of Action
It has been implicated in the temporal patterns of ketometabolism in cerebral microdialysis fluids of patients with traumatic brain injury , underscoring its significance in biochemical pathways within the brain.
Action Environment
It is known that certain conditions, such as lactation, are associated with elevated catabolism of bcaas in mammary glands to produce this compound .
Biochemical Analysis
Biochemical Properties
4-Methyl-2-oxopentanoic acid is a key intermediate in the catabolism of leucine. It is produced by the transamination of leucine and subsequently undergoes oxidative decarboxylation to form isovaleryl-CoA. This compound interacts with several enzymes, including branched-chain amino acid aminotransferase and branched-chain α-keto acid dehydrogenase complex. These interactions are crucial for the proper metabolism of branched-chain amino acids and energy production .
Cellular Effects
This compound influences various cellular processes, including cell signaling pathways, gene expression, and cellular metabolism. It has been shown to affect the mTOR signaling pathway, which is involved in cell growth and proliferation. Additionally, this compound can modulate the expression of genes related to amino acid metabolism and energy production, thereby impacting overall cellular function .
Molecular Mechanism
At the molecular level, this compound exerts its effects through binding interactions with specific enzymes and proteins. It acts as a substrate for branched-chain amino acid aminotransferase, facilitating the transfer of amino groups. Furthermore, it can inhibit or activate certain enzymes involved in amino acid metabolism, leading to changes in gene expression and metabolic flux .
Temporal Effects in Laboratory Settings
In laboratory settings, the effects of this compound can vary over time. The compound is relatively stable under standard conditions, but it can degrade under extreme pH or temperature conditions. Long-term studies have shown that prolonged exposure to this compound can lead to alterations in cellular function, including changes in metabolic pathways and gene expression .
Dosage Effects in Animal Models
The effects of this compound in animal models are dose-dependent. At low doses, it can promote normal metabolic function and energy production. At high doses, it may exhibit toxic effects, including neurotoxicity and disruption of normal cellular processes. Threshold effects have been observed, where a specific dosage range is required to achieve the desired biochemical effects without causing adverse reactions .
Metabolic Pathways
This compound is involved in several metabolic pathways, including the degradation of branched-chain amino acids and the biosynthesis of other metabolites. It interacts with enzymes such as branched-chain amino acid aminotransferase and branched-chain α-keto acid dehydrogenase complex, which are essential for its metabolism. These interactions influence metabolic flux and the levels of various metabolites within the cell .
Transport and Distribution
Within cells and tissues, this compound is transported and distributed through specific transporters and binding proteins. These transporters facilitate its movement across cellular membranes, ensuring its proper localization and accumulation. The distribution of this compound can affect its activity and function within different cellular compartments .
Subcellular Localization
The subcellular localization of this compound is influenced by targeting signals and post-translational modifications. It is primarily localized in the mitochondria, where it participates in the catabolism of branched-chain amino acids. The specific localization of this compound is crucial for its activity and function, as it ensures its interaction with the appropriate enzymes and metabolic pathways .
Preparation Methods
Synthetic Routes and Reaction Conditions: Alpha-Ketoisocaproic acid can be synthesized through the transamination of leucine. The enzyme branched-chain amino acid aminotransferase catalyzes the transfer of the amine group from leucine to alpha-ketoglutarate, forming alpha-Ketoisocaproic acid and glutamate .
Industrial Production Methods: Industrial production of alpha-Ketoisocaproic acid typically involves the fermentation of leucine using specific strains of bacteria or yeast that express the necessary enzymes for the transamination process. The fermentation broth is then processed to isolate and purify the compound .
Chemical Reactions Analysis
Types of Reactions: Alpha-Ketoisocaproic acid undergoes several types of chemical reactions, including:
Oxidation: It can be oxidized to produce various metabolites, such as isovaleryl-CoA.
Reduction: It can be reduced to form hydroxyisocaproic acid.
Transamination: It can participate in transamination reactions to form other amino acids.
Common Reagents and Conditions:
Oxidation: Common oxidizing agents include potassium permanganate and hydrogen peroxide.
Reduction: Reducing agents such as sodium borohydride or lithium aluminum hydride are used.
Transamination: This reaction typically requires alpha-ketoglutarate and the enzyme branched-chain amino acid aminotransferase.
Major Products:
Oxidation: Isovaleryl-CoA
Reduction: Hydroxyisocaproic acid
Transamination: Glutamate and other amino acids
Scientific Research Applications
Alpha-Ketoisocaproic acid has several scientific research applications:
Chemistry: It is used as a precursor in the synthesis of various organic compounds.
Biology: It is studied for its role in amino acid metabolism and its effects on cellular processes.
Medicine: It is used in nutritional therapy for managing chronic renal failure and acute renal dysfunction.
Industry: It is used in the production of dietary supplements and as a biochemical reagent.
Comparison with Similar Compounds
- Alpha-Ketoisovaleric acid
- Alpha-Ketobutyric acid
- Alpha-Ketoglutaric acid
- Alpha-Keto-beta-methylvaleric acid
Comparison: Alpha-Ketoisocaproic acid is unique due to its specific role in leucine metabolism. While other alpha-keto acids are involved in different metabolic pathways, alpha-Ketoisocaproic acid is specifically linked to the degradation of leucine and the production of isovaleryl-CoA. This specificity makes it a critical compound in studies related to leucine metabolism and its associated biological effects .
Properties
IUPAC Name |
4-methyl-2-oxopentanoic acid | |
---|---|---|
Source | PubChem | |
URL | https://pubchem.ncbi.nlm.nih.gov | |
Description | Data deposited in or computed by PubChem | |
InChI |
InChI=1S/C6H10O3/c1-4(2)3-5(7)6(8)9/h4H,3H2,1-2H3,(H,8,9) | |
Source | PubChem | |
URL | https://pubchem.ncbi.nlm.nih.gov | |
Description | Data deposited in or computed by PubChem | |
InChI Key |
BKAJNAXTPSGJCU-UHFFFAOYSA-N | |
Source | PubChem | |
URL | https://pubchem.ncbi.nlm.nih.gov | |
Description | Data deposited in or computed by PubChem | |
Canonical SMILES |
CC(C)CC(=O)C(=O)O | |
Source | PubChem | |
URL | https://pubchem.ncbi.nlm.nih.gov | |
Description | Data deposited in or computed by PubChem | |
Molecular Formula |
C6H10O3 | |
Source | PubChem | |
URL | https://pubchem.ncbi.nlm.nih.gov | |
Description | Data deposited in or computed by PubChem | |
Related CAS |
4502-00-5 (hydrochloride salt), 51828-95-6 (calcium salt) | |
Record name | alpha-Ketoisocaproic acid | |
Source | ChemIDplus | |
URL | https://pubchem.ncbi.nlm.nih.gov/substance/?source=chemidplus&sourceid=0000816660 | |
Description | ChemIDplus is a free, web search system that provides access to the structure and nomenclature authority files used for the identification of chemical substances cited in National Library of Medicine (NLM) databases, including the TOXNET system. | |
DSSTOX Substance ID |
DTXSID6061157 | |
Record name | alpha-Ketoisocaproic acid | |
Source | EPA DSSTox | |
URL | https://comptox.epa.gov/dashboard/DTXSID6061157 | |
Description | DSSTox provides a high quality public chemistry resource for supporting improved predictive toxicology. | |
Molecular Weight |
130.14 g/mol | |
Source | PubChem | |
URL | https://pubchem.ncbi.nlm.nih.gov | |
Description | Data deposited in or computed by PubChem | |
Physical Description |
Liquid, Pale yellow liquid; fruity aroma | |
Record name | Ketoleucine | |
Source | Human Metabolome Database (HMDB) | |
URL | http://www.hmdb.ca/metabolites/HMDB0000695 | |
Description | The Human Metabolome Database (HMDB) is a freely available electronic database containing detailed information about small molecule metabolites found in the human body. | |
Explanation | HMDB is offered to the public as a freely available resource. Use and re-distribution of the data, in whole or in part, for commercial purposes requires explicit permission of the authors and explicit acknowledgment of the source material (HMDB) and the original publication (see the HMDB citing page). We ask that users who download significant portions of the database cite the HMDB paper in any resulting publications. | |
Record name | 4-Methyl-2-oxopentanoic acid | |
Source | Joint FAO/WHO Expert Committee on Food Additives (JECFA) | |
URL | https://www.fao.org/food/food-safety-quality/scientific-advice/jecfa/jecfa-flav/details/en/c/454/ | |
Description | The flavoring agent databse provides the most recent specifications for flavorings evaluated by JECFA. | |
Explanation | Permission from WHO is not required for the use of WHO materials issued under the Creative Commons Attribution-NonCommercial-ShareAlike 3.0 Intergovernmental Organization (CC BY-NC-SA 3.0 IGO) licence. | |
Solubility |
32 mg/mL, Soluble in water, Soluble (in ethanol) | |
Record name | Ketoleucine | |
Source | Human Metabolome Database (HMDB) | |
URL | http://www.hmdb.ca/metabolites/HMDB0000695 | |
Description | The Human Metabolome Database (HMDB) is a freely available electronic database containing detailed information about small molecule metabolites found in the human body. | |
Explanation | HMDB is offered to the public as a freely available resource. Use and re-distribution of the data, in whole or in part, for commercial purposes requires explicit permission of the authors and explicit acknowledgment of the source material (HMDB) and the original publication (see the HMDB citing page). We ask that users who download significant portions of the database cite the HMDB paper in any resulting publications. | |
Record name | 4-Methyl-2-oxopentanoic acid | |
Source | Joint FAO/WHO Expert Committee on Food Additives (JECFA) | |
URL | https://www.fao.org/food/food-safety-quality/scientific-advice/jecfa/jecfa-flav/details/en/c/454/ | |
Description | The flavoring agent databse provides the most recent specifications for flavorings evaluated by JECFA. | |
Explanation | Permission from WHO is not required for the use of WHO materials issued under the Creative Commons Attribution-NonCommercial-ShareAlike 3.0 Intergovernmental Organization (CC BY-NC-SA 3.0 IGO) licence. | |
Density |
1.053-1.058 | |
Record name | 4-Methyl-2-oxopentanoic acid | |
Source | Joint FAO/WHO Expert Committee on Food Additives (JECFA) | |
URL | https://www.fao.org/food/food-safety-quality/scientific-advice/jecfa/jecfa-flav/details/en/c/454/ | |
Description | The flavoring agent databse provides the most recent specifications for flavorings evaluated by JECFA. | |
Explanation | Permission from WHO is not required for the use of WHO materials issued under the Creative Commons Attribution-NonCommercial-ShareAlike 3.0 Intergovernmental Organization (CC BY-NC-SA 3.0 IGO) licence. | |
CAS No. |
816-66-0 | |
Record name | 4-Methyl-2-oxovaleric acid | |
Source | CAS Common Chemistry | |
URL | https://commonchemistry.cas.org/detail?cas_rn=816-66-0 | |
Description | CAS Common Chemistry is an open community resource for accessing chemical information. Nearly 500,000 chemical substances from CAS REGISTRY cover areas of community interest, including common and frequently regulated chemicals, and those relevant to high school and undergraduate chemistry classes. This chemical information, curated by our expert scientists, is provided in alignment with our mission as a division of the American Chemical Society. | |
Explanation | The data from CAS Common Chemistry is provided under a CC-BY-NC 4.0 license, unless otherwise stated. | |
Record name | alpha-Ketoisocaproic acid | |
Source | ChemIDplus | |
URL | https://pubchem.ncbi.nlm.nih.gov/substance/?source=chemidplus&sourceid=0000816660 | |
Description | ChemIDplus is a free, web search system that provides access to the structure and nomenclature authority files used for the identification of chemical substances cited in National Library of Medicine (NLM) databases, including the TOXNET system. | |
Record name | alpha-Ketoisocaproic acid | |
Source | DrugBank | |
URL | https://www.drugbank.ca/drugs/DB03229 | |
Description | The DrugBank database is a unique bioinformatics and cheminformatics resource that combines detailed drug (i.e. chemical, pharmacological and pharmaceutical) data with comprehensive drug target (i.e. sequence, structure, and pathway) information. | |
Explanation | Creative Common's Attribution-NonCommercial 4.0 International License (http://creativecommons.org/licenses/by-nc/4.0/legalcode) | |
Record name | Pentanoic acid, 4-methyl-2-oxo- | |
Source | EPA Chemicals under the TSCA | |
URL | https://www.epa.gov/chemicals-under-tsca | |
Description | EPA Chemicals under the Toxic Substances Control Act (TSCA) collection contains information on chemicals and their regulations under TSCA, including non-confidential content from the TSCA Chemical Substance Inventory and Chemical Data Reporting. | |
Record name | alpha-Ketoisocaproic acid | |
Source | EPA DSSTox | |
URL | https://comptox.epa.gov/dashboard/DTXSID6061157 | |
Description | DSSTox provides a high quality public chemistry resource for supporting improved predictive toxicology. | |
Record name | 4-methyl-2-oxovaleric acid | |
Source | European Chemicals Agency (ECHA) | |
URL | https://echa.europa.eu/substance-information/-/substanceinfo/100.011.304 | |
Description | The European Chemicals Agency (ECHA) is an agency of the European Union which is the driving force among regulatory authorities in implementing the EU's groundbreaking chemicals legislation for the benefit of human health and the environment as well as for innovation and competitiveness. | |
Explanation | Use of the information, documents and data from the ECHA website is subject to the terms and conditions of this Legal Notice, and subject to other binding limitations provided for under applicable law, the information, documents and data made available on the ECHA website may be reproduced, distributed and/or used, totally or in part, for non-commercial purposes provided that ECHA is acknowledged as the source: "Source: European Chemicals Agency, http://echa.europa.eu/". Such acknowledgement must be included in each copy of the material. ECHA permits and encourages organisations and individuals to create links to the ECHA website under the following cumulative conditions: Links can only be made to webpages that provide a link to the Legal Notice page. | |
Record name | .ALPHA.-KETOISOCAPROIC ACID | |
Source | FDA Global Substance Registration System (GSRS) | |
URL | https://gsrs.ncats.nih.gov/ginas/app/beta/substances/4GUJ8AH400 | |
Description | The FDA Global Substance Registration System (GSRS) enables the efficient and accurate exchange of information on what substances are in regulated products. Instead of relying on names, which vary across regulatory domains, countries, and regions, the GSRS knowledge base makes it possible for substances to be defined by standardized, scientific descriptions. | |
Explanation | Unless otherwise noted, the contents of the FDA website (www.fda.gov), both text and graphics, are not copyrighted. They are in the public domain and may be republished, reprinted and otherwise used freely by anyone without the need to obtain permission from FDA. Credit to the U.S. Food and Drug Administration as the source is appreciated but not required. | |
Record name | Ketoleucine | |
Source | Human Metabolome Database (HMDB) | |
URL | http://www.hmdb.ca/metabolites/HMDB0000695 | |
Description | The Human Metabolome Database (HMDB) is a freely available electronic database containing detailed information about small molecule metabolites found in the human body. | |
Explanation | HMDB is offered to the public as a freely available resource. Use and re-distribution of the data, in whole or in part, for commercial purposes requires explicit permission of the authors and explicit acknowledgment of the source material (HMDB) and the original publication (see the HMDB citing page). We ask that users who download significant portions of the database cite the HMDB paper in any resulting publications. | |
Melting Point |
8 - 10 °C | |
Record name | Ketoleucine | |
Source | Human Metabolome Database (HMDB) | |
URL | http://www.hmdb.ca/metabolites/HMDB0000695 | |
Description | The Human Metabolome Database (HMDB) is a freely available electronic database containing detailed information about small molecule metabolites found in the human body. | |
Explanation | HMDB is offered to the public as a freely available resource. Use and re-distribution of the data, in whole or in part, for commercial purposes requires explicit permission of the authors and explicit acknowledgment of the source material (HMDB) and the original publication (see the HMDB citing page). We ask that users who download significant portions of the database cite the HMDB paper in any resulting publications. | |
Synthesis routes and methods
Procedure details
Retrosynthesis Analysis
AI-Powered Synthesis Planning: Our tool employs the Template_relevance Pistachio, Template_relevance Bkms_metabolic, Template_relevance Pistachio_ringbreaker, Template_relevance Reaxys, Template_relevance Reaxys_biocatalysis model, leveraging a vast database of chemical reactions to predict feasible synthetic routes.
One-Step Synthesis Focus: Specifically designed for one-step synthesis, it provides concise and direct routes for your target compounds, streamlining the synthesis process.
Accurate Predictions: Utilizing the extensive PISTACHIO, BKMS_METABOLIC, PISTACHIO_RINGBREAKER, REAXYS, REAXYS_BIOCATALYSIS database, our tool offers high-accuracy predictions, reflecting the latest in chemical research and data.
Strategy Settings
Precursor scoring | Relevance Heuristic |
---|---|
Min. plausibility | 0.01 |
Model | Template_relevance |
Template Set | Pistachio/Bkms_metabolic/Pistachio_ringbreaker/Reaxys/Reaxys_biocatalysis |
Top-N result to add to graph | 6 |
Feasible Synthetic Routes
Disclaimer and Information on In-Vitro Research Products
Please be aware that all articles and product information presented on BenchChem are intended solely for informational purposes. The products available for purchase on BenchChem are specifically designed for in-vitro studies, which are conducted outside of living organisms. In-vitro studies, derived from the Latin term "in glass," involve experiments performed in controlled laboratory settings using cells or tissues. It is important to note that these products are not categorized as medicines or drugs, and they have not received approval from the FDA for the prevention, treatment, or cure of any medical condition, ailment, or disease. We must emphasize that any form of bodily introduction of these products into humans or animals is strictly prohibited by law. It is essential to adhere to these guidelines to ensure compliance with legal and ethical standards in research and experimentation.