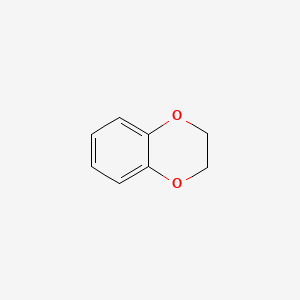
1,4-Benzodioxane
Overview
Description
1,4-Benzodioxane is an organic compound characterized by a benzene ring fused with a dioxane ring. This structure forms a bicyclic system with two oxygen atoms at the 1 and 4 positions of the dioxane ring. It is a versatile scaffold in medicinal chemistry and is found in various natural products and synthetic compounds.
Mechanism of Action
Target of Action
1,4-Benzodioxane is a lignan-derived natural product that exhibits a diverse array of biological activities . It has been shown to have significant interactions with various targets, including Gram-negative bacteria strains . The compound’s primary targets are believed to be associated with its antimicrobial activity .
Mode of Action
The mode of action of this compound involves its interaction with these targets, leading to changes in their function. For instance, it has been found to demonstrate higher antibacterial activity against Gram-negative bacteria strains than Gram-positive bacteria strains . The compound’s interaction with its targets is believed to inhibit their normal function, thereby exerting its biological effects .
Biochemical Pathways
This compound oxyneolignans are believed to be formed by the phenolic coupling of two C6C3 units through a three-step process . This process involves (A) enzymatically-promoted phenol oxidation of the two phenylpropane units, (B) O-β coupling of the resulting phenoxy radicals, and © cyclisation of the quinone methide system . These biochemical pathways are crucial for the compound’s biological activity.
Result of Action
The molecular and cellular effects of this compound’s action are diverse, reflecting its wide range of biological activities. For instance, it has been found to exhibit significant antibacterial activity . Additionally, it has been shown to have hepaprotective activities, prostaglandin I2 inducer activity, neurotrophic (ChAT) activity, insecticidal activity, COX-1 and COX-2 inhibition, anti-angiogenic activity, MDR pump inhibition activity, anti-cancer activity, and anti-oxidant activity .
Biochemical Analysis
Biochemical Properties
1,4-Benzodioxane plays a significant role in biochemical reactions. It interacts with various enzymes, proteins, and other biomolecules. For instance, this compound derivatives have been shown to inhibit the enzyme FtsZ, which is crucial for bacterial cell division . This interaction disrupts the formation of the Z-ring, leading to the inhibition of bacterial cell division. Additionally, this compound has been found to interact with adrenergic and serotoninergic receptors, influencing neurotransmission and exhibiting potential as a therapeutic agent for neurological disorders .
Cellular Effects
This compound affects various types of cells and cellular processes. It has been observed to influence cell signaling pathways, gene expression, and cellular metabolism. For example, this compound derivatives have shown antimicrobial activity by targeting bacterial cell division proteins . In mammalian cells, this compound can modulate neurotransmitter release by interacting with adrenergic and serotoninergic receptors, thereby affecting neuronal signaling and potentially offering therapeutic benefits for conditions such as depression and anxiety .
Molecular Mechanism
The molecular mechanism of this compound involves its binding interactions with biomolecules, enzyme inhibition, and changes in gene expression. This compound derivatives inhibit the FtsZ enzyme by binding to its active site, preventing the polymerization required for bacterial cell division . Additionally, this compound can act as an antagonist at adrenergic and serotoninergic receptors, blocking the binding of natural ligands and modulating receptor activity . These interactions result in altered cellular responses and therapeutic effects.
Temporal Effects in Laboratory Settings
In laboratory settings, the effects of this compound have been studied over time to understand its stability, degradation, and long-term impact on cellular function. Studies have shown that this compound is relatively stable under standard laboratory conditions In vitro and in vivo studies have indicated that prolonged exposure to this compound can lead to sustained inhibition of bacterial growth and modulation of neurotransmitter systems .
Dosage Effects in Animal Models
The effects of this compound vary with different dosages in animal models. At lower doses, this compound derivatives have shown antimicrobial activity without significant toxicity . At higher doses, toxic effects such as hepatotoxicity and neurotoxicity have been observed . These findings highlight the importance of determining the optimal dosage for therapeutic applications to minimize adverse effects while maximizing efficacy.
Metabolic Pathways
This compound is involved in various metabolic pathways. It interacts with enzymes and cofactors that facilitate its biotransformation. For instance, this compound can undergo hydroxylation and conjugation reactions, leading to the formation of metabolites that are excreted from the body . These metabolic pathways are crucial for understanding the pharmacokinetics and potential drug interactions of this compound.
Transport and Distribution
The transport and distribution of this compound within cells and tissues involve interactions with transporters and binding proteins. This compound can be transported across cell membranes via passive diffusion or facilitated transport mechanisms . Once inside the cell, it can bind to specific proteins that influence its localization and accumulation. These interactions are essential for determining the bioavailability and therapeutic potential of this compound.
Subcellular Localization
The subcellular localization of this compound affects its activity and function. This compound can be directed to specific cellular compartments or organelles through targeting signals or post-translational modifications . For example, this compound derivatives may localize to the endoplasmic reticulum or mitochondria, where they exert their effects on cellular processes. Understanding the subcellular localization of this compound is crucial for elucidating its mechanism of action and optimizing its therapeutic applications.
Preparation Methods
Synthetic Routes and Reaction Conditions: 1,4-Benzodioxane can be synthesized through several methods:
Oxidative Dimerization: This method involves the oxidative coupling of phenolic compounds.
Phenolate Substitution: This involves the substitution of a bromine atom in an α-bromophenone with a phenolate ion.
Condensation of Epoxide Precursors: This method uses epoxide precursors that undergo condensation reactions to form the this compound structure.
Transition Metal-Assisted Methods: These methods involve the use of transition metals like palladium or nickel to catalyze the formation of the this compound ring.
Mitsunobu Coupling: This method involves the coupling of phenols and chiral alcohols using Mitsunobu reagents.
Industrial Production Methods: Industrial production of this compound often involves scalable synthetic routes such as transition metal-catalyzed reactions and oxidative dimerization, which can be optimized for large-scale production .
Chemical Reactions Analysis
1,4-Benzodioxane undergoes various chemical reactions, including:
Oxidation: It can be oxidized to form quinone derivatives.
Reduction: Reduction reactions can convert this compound to its dihydro derivatives.
Substitution: Electrophilic and nucleophilic substitution reactions can introduce various functional groups into the benzodioxane ring.
Major Products:
Oxidation Products: Quinone derivatives.
Reduction Products: Dihydro-1,4-benzodioxane.
Substitution Products: Functionalized benzodioxane derivatives with various substituents.
Scientific Research Applications
1,4-Benzodioxane has a wide range of applications in scientific research:
Comparison with Similar Compounds
1,4-Benzodioxane is unique compared to other benzodioxane isomers due to its specific ring structure and biological activities. Similar compounds include:
1,2-Benzodioxane: Differing in the position of oxygen atoms, leading to different chemical properties and biological activities.
1,3-Benzodioxane: Another isomer with distinct reactivity and applications.
Derivatives: Compounds like eltoprazine, fluparoxan, and piperoxan, which are used in pharmaceuticals.
This compound stands out due to its versatility and wide range of applications in various fields, making it a valuable compound in both research and industry.
Properties
IUPAC Name |
2,3-dihydro-1,4-benzodioxine | |
---|---|---|
Source | PubChem | |
URL | https://pubchem.ncbi.nlm.nih.gov | |
Description | Data deposited in or computed by PubChem | |
InChI |
InChI=1S/C8H8O2/c1-2-4-8-7(3-1)9-5-6-10-8/h1-4H,5-6H2 | |
Source | PubChem | |
URL | https://pubchem.ncbi.nlm.nih.gov | |
Description | Data deposited in or computed by PubChem | |
InChI Key |
BNBQRQQYDMDJAH-UHFFFAOYSA-N | |
Source | PubChem | |
URL | https://pubchem.ncbi.nlm.nih.gov | |
Description | Data deposited in or computed by PubChem | |
Canonical SMILES |
C1COC2=CC=CC=C2O1 | |
Source | PubChem | |
URL | https://pubchem.ncbi.nlm.nih.gov | |
Description | Data deposited in or computed by PubChem | |
Molecular Formula |
C8H8O2 | |
Source | PubChem | |
URL | https://pubchem.ncbi.nlm.nih.gov | |
Description | Data deposited in or computed by PubChem | |
DSSTOX Substance ID |
DTXSID8075419 | |
Record name | 1,4-Benzodioxan | |
Source | EPA DSSTox | |
URL | https://comptox.epa.gov/dashboard/DTXSID8075419 | |
Description | DSSTox provides a high quality public chemistry resource for supporting improved predictive toxicology. | |
Molecular Weight |
136.15 g/mol | |
Source | PubChem | |
URL | https://pubchem.ncbi.nlm.nih.gov | |
Description | Data deposited in or computed by PubChem | |
CAS No. |
493-09-4 | |
Record name | 1,4-Benzodioxan | |
Source | CAS Common Chemistry | |
URL | https://commonchemistry.cas.org/detail?cas_rn=493-09-4 | |
Description | CAS Common Chemistry is an open community resource for accessing chemical information. Nearly 500,000 chemical substances from CAS REGISTRY cover areas of community interest, including common and frequently regulated chemicals, and those relevant to high school and undergraduate chemistry classes. This chemical information, curated by our expert scientists, is provided in alignment with our mission as a division of the American Chemical Society. | |
Explanation | The data from CAS Common Chemistry is provided under a CC-BY-NC 4.0 license, unless otherwise stated. | |
Record name | 1,4-Benzodioxan | |
Source | ChemIDplus | |
URL | https://pubchem.ncbi.nlm.nih.gov/substance/?source=chemidplus&sourceid=0000493094 | |
Description | ChemIDplus is a free, web search system that provides access to the structure and nomenclature authority files used for the identification of chemical substances cited in National Library of Medicine (NLM) databases, including the TOXNET system. | |
Record name | 1,4-Benzodioxane | |
Source | DTP/NCI | |
URL | https://dtp.cancer.gov/dtpstandard/servlet/dwindex?searchtype=NSC&outputformat=html&searchlist=406705 | |
Description | The NCI Development Therapeutics Program (DTP) provides services and resources to the academic and private-sector research communities worldwide to facilitate the discovery and development of new cancer therapeutic agents. | |
Explanation | Unless otherwise indicated, all text within NCI products is free of copyright and may be reused without our permission. Credit the National Cancer Institute as the source. | |
Record name | 1,4-Benzodioxan | |
Source | EPA DSSTox | |
URL | https://comptox.epa.gov/dashboard/DTXSID8075419 | |
Description | DSSTox provides a high quality public chemistry resource for supporting improved predictive toxicology. | |
Record name | 1,4-benzodioxane | |
Source | European Chemicals Agency (ECHA) | |
URL | https://echa.europa.eu/substance-information/-/substanceinfo/100.007.069 | |
Description | The European Chemicals Agency (ECHA) is an agency of the European Union which is the driving force among regulatory authorities in implementing the EU's groundbreaking chemicals legislation for the benefit of human health and the environment as well as for innovation and competitiveness. | |
Explanation | Use of the information, documents and data from the ECHA website is subject to the terms and conditions of this Legal Notice, and subject to other binding limitations provided for under applicable law, the information, documents and data made available on the ECHA website may be reproduced, distributed and/or used, totally or in part, for non-commercial purposes provided that ECHA is acknowledged as the source: "Source: European Chemicals Agency, http://echa.europa.eu/". Such acknowledgement must be included in each copy of the material. ECHA permits and encourages organisations and individuals to create links to the ECHA website under the following cumulative conditions: Links can only be made to webpages that provide a link to the Legal Notice page. | |
Record name | 1,4-BENZODIOXAN | |
Source | FDA Global Substance Registration System (GSRS) | |
URL | https://gsrs.ncats.nih.gov/ginas/app/beta/substances/ZC5YP57PSW | |
Description | The FDA Global Substance Registration System (GSRS) enables the efficient and accurate exchange of information on what substances are in regulated products. Instead of relying on names, which vary across regulatory domains, countries, and regions, the GSRS knowledge base makes it possible for substances to be defined by standardized, scientific descriptions. | |
Explanation | Unless otherwise noted, the contents of the FDA website (www.fda.gov), both text and graphics, are not copyrighted. They are in the public domain and may be republished, reprinted and otherwise used freely by anyone without the need to obtain permission from FDA. Credit to the U.S. Food and Drug Administration as the source is appreciated but not required. | |
Synthesis routes and methods I
Procedure details
Synthesis routes and methods II
Procedure details
Retrosynthesis Analysis
AI-Powered Synthesis Planning: Our tool employs the Template_relevance Pistachio, Template_relevance Bkms_metabolic, Template_relevance Pistachio_ringbreaker, Template_relevance Reaxys, Template_relevance Reaxys_biocatalysis model, leveraging a vast database of chemical reactions to predict feasible synthetic routes.
One-Step Synthesis Focus: Specifically designed for one-step synthesis, it provides concise and direct routes for your target compounds, streamlining the synthesis process.
Accurate Predictions: Utilizing the extensive PISTACHIO, BKMS_METABOLIC, PISTACHIO_RINGBREAKER, REAXYS, REAXYS_BIOCATALYSIS database, our tool offers high-accuracy predictions, reflecting the latest in chemical research and data.
Strategy Settings
Precursor scoring | Relevance Heuristic |
---|---|
Min. plausibility | 0.01 |
Model | Template_relevance |
Template Set | Pistachio/Bkms_metabolic/Pistachio_ringbreaker/Reaxys/Reaxys_biocatalysis |
Top-N result to add to graph | 6 |
Feasible Synthetic Routes
Q1: What is 1,4-benzodioxane and what makes it interesting for medicinal chemistry?
A1: this compound is a heterocyclic organic compound that serves as a valuable scaffold in medicinal chemistry. Its derivatives exhibit a wide array of biological activities, making them attractive targets for drug discovery and development.
Q2: Can you provide specific examples of how this compound derivatives interact with their biological targets and their downstream effects?
A2: * α-Adrenergic Antagonists: Certain enantiomers of 2-[[[2-(2,6-dimethoxyphenoxy)ethyl]amino]methyl]-1,4-benzodioxane (WB-4101) exhibit potent competitive antagonism at α-adrenergic receptors, particularly the (2S)-enantiomer, with a pA2 = 9.0. [] This antagonism translates to a relaxation of smooth muscle and a reduction in blood pressure.* Antiviral Activity: Eusiderins A, B, G, and M, deallyl eusiderin A, and nitidanin, this compound neolignans, have demonstrated greater antiviral activity compared to the well-known silybin flavonolignans. [] These compounds appear to exert their antiviral effects through mechanisms distinct from those of the flavonolignans, which possess a chromanone motif not found in the eusiderins.* Acetylcholinesterase Inhibition: Piperazine derivatives containing the this compound moiety have demonstrated inhibitory activity against human acetylcholinesterase. [] These compounds, by inhibiting the breakdown of acetylcholine, could potentially serve as therapeutic agents for Alzheimer's disease.
Q3: What is the molecular formula and weight of this compound?
A3: The molecular formula of this compound is C8H8O2, and its molecular weight is 136.15 g/mol.
Q4: Are there any spectroscopic data available to characterize this compound and its derivatives?
A4: Yes, various spectroscopic techniques are employed to characterize this compound derivatives. These include:
- NMR Spectroscopy (1H NMR, 13C NMR, HSQC, HMBC): Used to determine the structure and regiochemistry of synthesized compounds, particularly for differentiating positional isomers. [, , , ]
- IR Spectroscopy (FT-IR): Provides information about functional groups present in the molecule. []
- Mass Spectrometry (LC-MS): Used to determine molecular weight and identify fragments for structural elucidation. []
- Circular Dichroism (CD): Employed to determine the absolute configuration of chiral this compound derivatives, particularly in comparison with natural products. [, ]
Q5: How does the structure of this compound derivatives influence their activity, potency, and selectivity?
A5: Structure-activity relationship (SAR) studies have revealed crucial insights into how modifications to the this compound scaffold affect biological activity:
- Substituent Effects: The type, position, and stereochemistry of substituents on the benzodioxane ring significantly influence its interactions with biological targets, leading to variations in potency and selectivity. [, , ]
- Chirality: Enantiomers of this compound derivatives often display distinct pharmacological profiles. For instance, the (2S)-enantiomer of WB-4101 exhibits significantly higher potency as an α-adrenergic antagonist compared to its (2R)-counterpart. []
- Ring Modifications: Replacing the this compound ring with a less conformationally constrained 1,4-dioxane ring can alter the binding affinity and selectivity towards different receptor systems, such as muscarinic receptors, α-adrenergic receptors, and 5-HT1A receptors. [, ]
Q6: Have any computational chemistry methods been used to study this compound derivatives?
A6: Yes, computational methods like molecular docking and quantitative structure-activity relationship (QSAR) studies have been employed.
- Molecular Docking: Docking studies help predict binding modes and interactions of this compound derivatives with their target proteins, aiding in the rational design of novel compounds. [, ]
- QSAR Modeling: QSAR models correlate the physicochemical properties of this compound derivatives with their biological activities, facilitating the prediction of activities for new compounds and guiding further optimization efforts. []
Q7: What are the known synthetic routes to this compound derivatives?
A7: Several synthetic strategies have been developed, including:
- Condensation Reactions: Reaction of catechol derivatives with 2,3-dibromopropionates in the presence of a base is a common method to construct the this compound ring. [, , ]
- Cyclization Reactions: Chiral 1,4-benzodioxanes can be synthesized through the sulfonylation of optically active triol derivatives followed by base-mediated cyclization. []
- Oxidative Coupling: Silver-catalyzed oxidative coupling of caffeic acid derivatives with suitable coupling partners provides regioselective access to 3-aryl-1,4-benzodioxanes. []
- Asymmetric Synthesis: Chiral auxiliaries, like ephedrine, have been utilized to achieve the asymmetric synthesis of 3-methyl-2-phenyl-1,4-benzodioxanes. []
Q8: Have any enzymatic methods been explored for the synthesis of chiral this compound derivatives?
A8: Yes, biocatalytic approaches employing nitrilases have been successfully utilized for the kinetic resolution of racemic 2-cyano-1,4-benzodioxanes. [] These enzymes catalyze the enantioselective hydrolysis of the nitrile group, yielding optically active this compound-2-carboxylic acids. [, ]
Q9: Are there any challenges associated with the stability and formulation of this compound derivatives?
A9: While specific stability data for all this compound derivatives is not provided in the provided research, general strategies to improve drug stability, solubility, and bioavailability are often applied. These may include:
Disclaimer and Information on In-Vitro Research Products
Please be aware that all articles and product information presented on BenchChem are intended solely for informational purposes. The products available for purchase on BenchChem are specifically designed for in-vitro studies, which are conducted outside of living organisms. In-vitro studies, derived from the Latin term "in glass," involve experiments performed in controlled laboratory settings using cells or tissues. It is important to note that these products are not categorized as medicines or drugs, and they have not received approval from the FDA for the prevention, treatment, or cure of any medical condition, ailment, or disease. We must emphasize that any form of bodily introduction of these products into humans or animals is strictly prohibited by law. It is essential to adhere to these guidelines to ensure compliance with legal and ethical standards in research and experimentation.