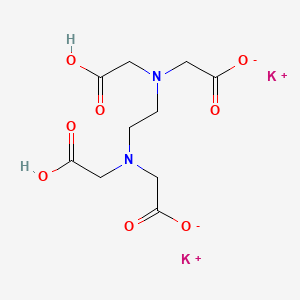
Glycine, N,N'-1,2-ethanediylbis[N-(carboxymethyl)-, dipotassium salt
Overview
Description
Glycine, N,N’-1,2-ethanediylbis[N-(carboxymethyl)-, dipotassium salt], also known as Ethylenediamine-N,N’-diacetic acid or EDDA, is a compound with the formula C6H12N2O4 . It has a molecular weight of 176.1705 .
Molecular Structure Analysis
The molecular structure of this compound consists of a central ethylenediamine moiety, with carboxymethyl groups attached to the nitrogen atoms . The 3D structure can be viewed using specific software .Scientific Research Applications
Hematology: Anticoagulant in Blood Analysis
Dipotassium EDTA is widely used as an anticoagulant in blood samples for hematological tests. It preserves blood cell morphology and inhibits the enzymes that cause blood clotting. This property is essential for accurate measurement of hematologic parameters such as platelet count, mean platelet volume (MPV), and mean platelet component (MPC), which are indicators of platelet activation . The use of Dipotassium EDTA ensures that the blood samples can be analyzed without the interference of clotting, providing reliable results for clinical diagnosis and management of diseases.
Aquaculture: Fish Hematology
In aquaculture research, Dipotassium EDTA serves as an anticoagulant for blood analysis of fish species like brown trout. It is crucial for obtaining reliable hematological values, which are affected by factors like the choice of anticoagulant. Studies have shown that Dipotassium EDTA-treated samples can be used to measure packed cell volume (PCV), hemoglobin concentration (HGB), and white blood cell count (WBC) without inducing hemolysis, although there is a tendency for red blood cells to swell, affecting certain measurements .
Clinical Chemistry: Platelet Activation Monitoring
Dipotassium EDTA is used in clinical chemistry to monitor platelet activation status. It is particularly useful in systems like the ADVIA® 120 Hematology System, where it helps in ensuring that platelets are sphered and their activation status is not altered artifactually in vitro. This application is significant for patients at risk of thrombotic diseases, as it aids in the clinical evaluation and management by providing insights into platelet behavior .
Pre-analytical Variability in Hematology Testing
The brand and type of Dipotassium EDTA vacuum tubes can be a source of pre-analytical variability in routine hematology testing. Research has indicated that different brands of tubes may affect the results of full blood cell counts and white blood cell differential analyses. Therefore, understanding the impact of Dipotassium EDTA on these tests is important for ensuring consistency and accuracy in hematological assessments .
Cosmetic Industry: Safety Assessment
Dipotassium EDTA is also assessed for its safety in cosmetic formulations. It is one of the various salts of EDTA evaluated by expert panels to determine its safety as used in cosmetics. The compound is found to be safe based on animal and clinical data, which is crucial for its application in cosmetic products .
Anticoagulant Comparison Studies
Studies comparing the efficacy of different anticoagulants often include Dipotassium EDTA. For example, its effects are compared with those of tripotassium EDTA and lithium heparin to determine the optimal conditions for blood analysis. Such studies help in refining laboratory protocols for better diagnostic outcomes .
Mechanism of Action
Target of Action
Dipotassium EDTA, also known as Ethylenediaminetetraacetic acid dipotassium salt, primarily targets metal ions in biological systems . It is a well-known chelating agent that binds to calcium, magnesium, and zinc ions . These ions play crucial roles in various biological processes, including enzymatic reactions and cell adhesion .
Mode of Action
Dipotassium EDTA interacts with its targets by forming stable, water-soluble complexes . This interaction inhibits the activity of metalloproteases, enzymes that require metal ions for their function . By complexing the metal ions, particularly the zinc ion at the active center of metalloproteases, Dipotassium EDTA controls the reactivity of these ions .
Biochemical Pathways
The chelation of metal ions by Dipotassium EDTA affects several biochemical pathways. For instance, it prevents the joining of cadherins between cells, which are calcium-dependent adhesion molecules . This action prevents cell clumping in liquid suspension cultures and aids in detaching adherent cells for passaging . In addition, the chelation of metal ions required for nucleases helps maintain DNA integrity during extraction processes .
Pharmacokinetics
While specific pharmacokinetic data for Dipotassium EDTA is limited, it is known that EDTA compounds are primarily eliminated by hepatic metabolism . Renal excretion is an insignificant elimination pathway for these compounds . The pharmacokinetics of EDTA compounds can be influenced by factors such as dose, route of administration, and individual patient characteristics.
Result of Action
The molecular and cellular effects of Dipotassium EDTA’s action are primarily due to its chelating properties. By binding to metal ions, it can inhibit metal-dependent enzymes, influence various biological processes, and maintain the integrity of DNA . In clinical laboratories, it is used to preserve blood samples for analysis by preventing coagulation .
Action Environment
The action, efficacy, and stability of Dipotassium EDTA can be influenced by various environmental factors. For instance, the pH of the solution can affect its chelating ability . Furthermore, the presence of other ions in the solution can compete with the target ions for chelation, potentially affecting the compound’s efficacy .
Safety and Hazards
properties
IUPAC Name |
dipotassium;2-[2-[bis(carboxymethyl)amino]ethyl-(carboxylatomethyl)amino]acetate | |
---|---|---|
Source | PubChem | |
URL | https://pubchem.ncbi.nlm.nih.gov | |
Description | Data deposited in or computed by PubChem | |
InChI |
InChI=1S/C10H16N2O8.2K/c13-7(14)3-11(4-8(15)16)1-2-12(5-9(17)18)6-10(19)20;;/h1-6H2,(H,13,14)(H,15,16)(H,17,18)(H,19,20);;/q;2*+1/p-2 | |
Source | PubChem | |
URL | https://pubchem.ncbi.nlm.nih.gov | |
Description | Data deposited in or computed by PubChem | |
InChI Key |
QLBHNVFOQLIYTH-UHFFFAOYSA-L | |
Source | PubChem | |
URL | https://pubchem.ncbi.nlm.nih.gov | |
Description | Data deposited in or computed by PubChem | |
Canonical SMILES |
C(CN(CC(=O)[O-])CC(=O)[O-])N(CC(=O)O)CC(=O)O.[K+].[K+] | |
Source | PubChem | |
URL | https://pubchem.ncbi.nlm.nih.gov | |
Description | Data deposited in or computed by PubChem | |
Molecular Formula |
C10H14K2N2O8 | |
Source | PubChem | |
URL | https://pubchem.ncbi.nlm.nih.gov | |
Description | Data deposited in or computed by PubChem | |
Molecular Weight |
368.42 g/mol | |
Source | PubChem | |
URL | https://pubchem.ncbi.nlm.nih.gov | |
Description | Data deposited in or computed by PubChem | |
Physical Description |
Liquid, White powder; [Sigma-Aldrich MSDS] | |
Record name | Glycine, N,N'-1,2-ethanediylbis[N-(carboxymethyl)-, potassium salt (1:2) | |
Source | EPA Chemicals under the TSCA | |
URL | https://www.epa.gov/chemicals-under-tsca | |
Description | EPA Chemicals under the Toxic Substances Control Act (TSCA) collection contains information on chemicals and their regulations under TSCA, including non-confidential content from the TSCA Chemical Substance Inventory and Chemical Data Reporting. | |
Record name | Dipotassium EDTA dihydrate | |
Source | Haz-Map, Information on Hazardous Chemicals and Occupational Diseases | |
URL | https://haz-map.com/Agents/14088 | |
Description | Haz-Map® is an occupational health database designed for health and safety professionals and for consumers seeking information about the adverse effects of workplace exposures to chemical and biological agents. | |
Explanation | Copyright (c) 2022 Haz-Map(R). All rights reserved. Unless otherwise indicated, all materials from Haz-Map are copyrighted by Haz-Map(R). No part of these materials, either text or image may be used for any purpose other than for personal use. Therefore, reproduction, modification, storage in a retrieval system or retransmission, in any form or by any means, electronic, mechanical or otherwise, for reasons other than personal use, is strictly prohibited without prior written permission. | |
Product Name |
Glycine, N,N'-1,2-ethanediylbis[N-(carboxymethyl)-, dipotassium salt | |
CAS RN |
2001-94-7, 25102-12-9 | |
Record name | Glycine, N,N'-1,2-ethanediylbis[N-(carboxymethyl)-, potassium salt (1:2) | |
Source | EPA Chemicals under the TSCA | |
URL | https://www.epa.gov/chemicals-under-tsca | |
Description | EPA Chemicals under the Toxic Substances Control Act (TSCA) collection contains information on chemicals and their regulations under TSCA, including non-confidential content from the TSCA Chemical Substance Inventory and Chemical Data Reporting. | |
Record name | Dipotassium dihydrogen ethylenediaminetetraacetate | |
Source | European Chemicals Agency (ECHA) | |
URL | https://echa.europa.eu/substance-information/-/substanceinfo/100.016.269 | |
Description | The European Chemicals Agency (ECHA) is an agency of the European Union which is the driving force among regulatory authorities in implementing the EU's groundbreaking chemicals legislation for the benefit of human health and the environment as well as for innovation and competitiveness. | |
Explanation | Use of the information, documents and data from the ECHA website is subject to the terms and conditions of this Legal Notice, and subject to other binding limitations provided for under applicable law, the information, documents and data made available on the ECHA website may be reproduced, distributed and/or used, totally or in part, for non-commercial purposes provided that ECHA is acknowledged as the source: "Source: European Chemicals Agency, http://echa.europa.eu/". Such acknowledgement must be included in each copy of the material. ECHA permits and encourages organisations and individuals to create links to the ECHA website under the following cumulative conditions: Links can only be made to webpages that provide a link to the Legal Notice page. | |
Record name | Dipotassium dihydrogen ethylenediaminetetraacetate dihydrate | |
Source | European Chemicals Agency (ECHA) | |
URL | https://echa.europa.eu/information-on-chemicals | |
Description | The European Chemicals Agency (ECHA) is an agency of the European Union which is the driving force among regulatory authorities in implementing the EU's groundbreaking chemicals legislation for the benefit of human health and the environment as well as for innovation and competitiveness. | |
Explanation | Use of the information, documents and data from the ECHA website is subject to the terms and conditions of this Legal Notice, and subject to other binding limitations provided for under applicable law, the information, documents and data made available on the ECHA website may be reproduced, distributed and/or used, totally or in part, for non-commercial purposes provided that ECHA is acknowledged as the source: "Source: European Chemicals Agency, http://echa.europa.eu/". Such acknowledgement must be included in each copy of the material. ECHA permits and encourages organisations and individuals to create links to the ECHA website under the following cumulative conditions: Links can only be made to webpages that provide a link to the Legal Notice page. | |
Retrosynthesis Analysis
AI-Powered Synthesis Planning: Our tool employs the Template_relevance Pistachio, Template_relevance Bkms_metabolic, Template_relevance Pistachio_ringbreaker, Template_relevance Reaxys, Template_relevance Reaxys_biocatalysis model, leveraging a vast database of chemical reactions to predict feasible synthetic routes.
One-Step Synthesis Focus: Specifically designed for one-step synthesis, it provides concise and direct routes for your target compounds, streamlining the synthesis process.
Accurate Predictions: Utilizing the extensive PISTACHIO, BKMS_METABOLIC, PISTACHIO_RINGBREAKER, REAXYS, REAXYS_BIOCATALYSIS database, our tool offers high-accuracy predictions, reflecting the latest in chemical research and data.
Strategy Settings
Precursor scoring | Relevance Heuristic |
---|---|
Min. plausibility | 0.01 |
Model | Template_relevance |
Template Set | Pistachio/Bkms_metabolic/Pistachio_ringbreaker/Reaxys/Reaxys_biocatalysis |
Top-N result to add to graph | 6 |
Feasible Synthetic Routes
Q & A
ANone: Dipotassium EDTA functions as a chelating agent by binding to metal ions, primarily calcium (Ca2+), through its four carboxylate and two amine groups. This binding forms a stable, water-soluble complex, effectively removing the metal ion from its biological or chemical environment.
ANone: By chelating calcium ions, Dipotassium EDTA prevents blood clotting []. This makes it a crucial additive in blood collection tubes used for hematological analysis. In other applications, this chelation disrupts calcium-dependent enzymatic activities and disrupts cell membrane stability.
ANone: The molecular formula of Dipotassium EDTA is C10H14K2N2O8, and its molecular weight is 370.45 g/mol.
ANone: While the provided research papers do not delve into detailed spectroscopic data, specific information can be found in chemical databases and publications focused on the structural characterization of EDTA salts.
ANone: Research indicates that using different brands of Dipotassium EDTA vacuum tubes can introduce clinically relevant variations in hematological parameters such as hematocrit, mean corpuscular volume, and platelet distribution width [].
ANone: Dipotassium EDTA offers superior stability for ascorbic acid analysis compared to lithium heparin or serum. Blood collected in EDTA tubes and promptly separated, deproteinized, and preserved yields the highest ascorbic acid levels [].
ANone: Dipotassium EDTA is not typically known for its catalytic properties. Its primary function is as a chelating agent, disrupting metal-dependent processes rather than catalyzing reactions.
ANone: While the provided research does not specifically mention computational studies on Dipotassium EDTA, such techniques are valuable for exploring the structural and binding properties of chelating agents.
ANone: Modifying the EDTA structure by altering the substituents on the nitrogen atoms or the length of the carbon chain can significantly impact its chelating affinity and selectivity for different metal ions.
ANone: C-peptide demonstrates better stability in separated serum compared to plasma in samples stored at 2-8°C for up to 7 days when collected with Dipotassium EDTA. Insulin exhibits greater stability in EDTA plasma than serum across various storage conditions [].
ANone: Delaying centrifugation significantly impacts C-peptide and insulin stability, particularly at room temperature. Immediate centrifugation and cold storage are crucial for preserving these analytes [].
ANone: The Cosmetic Ingredient Review Expert Panel concluded that EDTA and its salts, including Dipotassium EDTA, are safe for use in cosmetic formulations at typical concentrations [].
Disclaimer and Information on In-Vitro Research Products
Please be aware that all articles and product information presented on BenchChem are intended solely for informational purposes. The products available for purchase on BenchChem are specifically designed for in-vitro studies, which are conducted outside of living organisms. In-vitro studies, derived from the Latin term "in glass," involve experiments performed in controlled laboratory settings using cells or tissues. It is important to note that these products are not categorized as medicines or drugs, and they have not received approval from the FDA for the prevention, treatment, or cure of any medical condition, ailment, or disease. We must emphasize that any form of bodily introduction of these products into humans or animals is strictly prohibited by law. It is essential to adhere to these guidelines to ensure compliance with legal and ethical standards in research and experimentation.