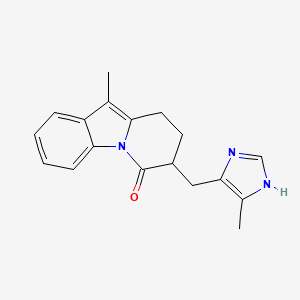
8,9-Dihydro-10-methyl-7-((5-methyl-1H-imidazol-4-yl)methyl)pyrido(1,2-a)indol-6(7H)-one
Overview
Description
8,9-Dihydro-10-methyl-7-((5-methyl-1H-imidazol-4-yl)methyl)pyrido(1,2-a)indol-6(7H)-one, also known as this compound, is a useful research compound. Its molecular formula is C18H19N3O and its molecular weight is 293.4 g/mol. The purity is usually 95%.
BenchChem offers high-quality this compound suitable for many research applications. Different packaging options are available to accommodate customers' requirements. Please inquire for more information about this compound including the price, delivery time, and more detailed information at info@benchchem.com.
Preparation Methods
Synthetic Routes and Reaction Conditions: The synthesis of FK 1052 involves several steps:
Condensation Reaction: The condensation of 10-methyl-6,7,8,9-tetrahydropyrido[1,2-a]indol-6-one with 5-methyl-1-(triphenylmethyl)-1H-imidazole-4-carboxaldehyde using butyllithium in tetrahydrofuran yields an intermediate compound.
Acetylation: This intermediate is then treated with acetic anhydride in pyridine to form an acetoxy derivative.
Elimination Reaction: The acetoxy derivative undergoes elimination of acetic acid in hot toluene to form a methylene derivative.
Hydrogenation: The methylene derivative is hydrogenated using hydrogen gas over palladium on carbon in dimethylformamide/ethanol to yield another intermediate.
Deprotection: Finally, the compound is deprotected in hot acetic acid/water to obtain FK 1052.
Industrial Production Methods: The industrial production of FK 1052 follows similar synthetic routes but is optimized for large-scale production. This involves the use of automated synthesis systems and controlled reaction conditions to ensure high yield and purity .
Chemical Reactions Analysis
Types of Reactions:
Oxidation: FK 1052 can undergo oxidation reactions, particularly at the indole ring.
Reduction: The compound can be reduced, especially during the hydrogenation step in its synthesis.
Substitution: FK 1052 can participate in substitution reactions, particularly at the imidazole ring.
Common Reagents and Conditions:
Oxidation: Common oxidizing agents include potassium permanganate and chromium trioxide.
Reduction: Hydrogen gas over palladium on carbon is commonly used.
Substitution: Reagents such as butyllithium and acetic anhydride are used.
Major Products: The major products formed from these reactions include various intermediates that eventually lead to the formation of FK 1052 .
Scientific Research Applications
Chemistry: FK 1052 is used as a model compound in studying dual receptor antagonists and their synthesis .
Biology: In biological research, FK 1052 is used to study the effects of serotonin receptor antagonism on various physiological processes .
Medicine: FK 1052 has been investigated for its potential use in treating chemotherapy-induced nausea and vomiting due to its dual receptor antagonistic properties .
Industry: In the pharmaceutical industry, FK 1052 is used in the development of new drugs targeting serotonin receptors .
Mechanism of Action
FK 1052 exerts its effects by antagonizing serotonin 3 and serotonin 4 receptors. This dual antagonism helps in reducing nausea and vomiting by blocking the action of serotonin at these receptors . The molecular targets include the serotonin receptors located in the gastrointestinal tract and the central nervous system .
Comparison with Similar Compounds
Ondansetron: A serotonin 3 receptor antagonist used to prevent nausea and vomiting.
Granisetron: Another serotonin 3 receptor antagonist with similar applications.
Uniqueness: FK 1052 is unique due to its dual antagonistic action on both serotonin 3 and serotonin 4 receptors, which provides a broader spectrum of activity compared to compounds like ondansetron and granisetron that primarily target serotonin 3 receptors .
Properties
CAS No. |
129299-72-5 |
---|---|
Molecular Formula |
C18H19N3O |
Molecular Weight |
293.4 g/mol |
IUPAC Name |
10-methyl-7-[(5-methyl-1H-imidazol-4-yl)methyl]-8,9-dihydro-7H-pyrido[1,2-a]indol-6-one |
InChI |
InChI=1S/C18H19N3O/c1-11-14-5-3-4-6-17(14)21-16(11)8-7-13(18(21)22)9-15-12(2)19-10-20-15/h3-6,10,13H,7-9H2,1-2H3,(H,19,20) |
InChI Key |
AEKQMJRJRAHOAP-UHFFFAOYSA-N |
SMILES |
CC1=C2CCC(C(=O)N2C3=CC=CC=C13)CC4=C(NC=N4)C |
Canonical SMILES |
CC1=C2CCC(C(=O)N2C3=CC=CC=C13)CC4=C(NC=N4)C |
Synonyms |
8,9-dihydro-10-dihydro-10-methyl-7-((5-methyl-4-imidazolyl)methyl)pyrido-(1-2a)-indol-6(7H)-one FK 1052 FK 1052, (+)-isomer FK 1052, (-)-isomer FK 1052, hydrochloride FK 1052, hydrochloride, (+)-isomer FK-1052 FK1052 |
Origin of Product |
United States |
Synthesis routes and methods I
Procedure details
Synthesis routes and methods II
Procedure details
Synthesis routes and methods III
Procedure details
Synthesis routes and methods IV
Procedure details
Synthesis routes and methods V
Procedure details
Disclaimer and Information on In-Vitro Research Products
Please be aware that all articles and product information presented on BenchChem are intended solely for informational purposes. The products available for purchase on BenchChem are specifically designed for in-vitro studies, which are conducted outside of living organisms. In-vitro studies, derived from the Latin term "in glass," involve experiments performed in controlled laboratory settings using cells or tissues. It is important to note that these products are not categorized as medicines or drugs, and they have not received approval from the FDA for the prevention, treatment, or cure of any medical condition, ailment, or disease. We must emphasize that any form of bodily introduction of these products into humans or animals is strictly prohibited by law. It is essential to adhere to these guidelines to ensure compliance with legal and ethical standards in research and experimentation.