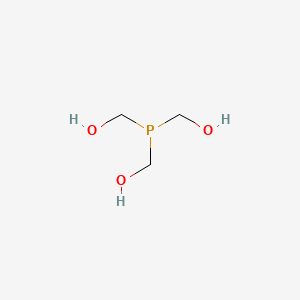
Tris(hydroxymethyl)phosphine
Overview
Description
Tris(hydroxymethyl)phosphine is an organophosphorus compound with the chemical formula P(CH₂OH)₃. It is a white solid that is multifunctional, consisting of three alcohol functional groups and a tertiary phosphine. This compound is known for its ability to form complexes with various metals and its reactivity in different chemical environments .
Mechanism of Action
Target of Action
Tris(hydroxymethyl)phosphine (THP) is an organophosphorus compound that primarily targets a variety of metals, forming complexes with them . These complexes are multifunctional, consisting of three alcohol functional groups and a tertiary phosphine .
Mode of Action
The interaction of THP with its targets involves the formation of complexes with metals . The gas-phase reaction of the synthesis of THP from phosphine and formaldehyde proceeds with the participation of unstable intermediates containing a three-membered ring . The transformation into final products includes the opening of the three-membered ring and intramolecular proton transfer .
Biochemical Pathways
The biochemical pathways affected by THP are primarily related to its role as a reducing agent. It has been demonstrated to be a versatile, water-soluble, and air-stable reducing agent, allowing for the rapid, irreversible reductive cleavage of disulfide bonds in both aqueous and buffered aqueous–organic media . This suggests that THP may play a role in redox reactions and disulfide bond formation in biological systems.
Pharmacokinetics
It is known that thp forms complexes that display some solubility in water and more so in methanol . This suggests that the compound may have good bioavailability due to its solubility.
Result of Action
The primary result of THP’s action is the formation of complexes with metals . These complexes are multifunctional, consisting of three alcohol functional groups and a tertiary phosphine . The compound decomposes violently to phosphine and formaldehyde upon attempted distillation . In air, it oxidizes to the oxide . Upon heating with hexamethylenetetramine, it converts to triazaphosphaadamantane .
Action Environment
The action of THP is influenced by environmental factors. For instance, in air, THP oxidizes to the oxide . The compound decomposes violently to phosphine and formaldehyde upon attempted distillation . This suggests that the stability and efficacy of THP can be influenced by factors such as oxygen levels and temperature.
Biochemical Analysis
Biochemical Properties
Tris(hydroxymethyl)phosphine plays a significant role in biochemical reactions due to its ability to form complexes with a variety of metals. These complexes exhibit solubility in water and methanol . The compound interacts with enzymes, proteins, and other biomolecules, forming stable complexes that can influence various biochemical pathways. For instance, it can act as a ligand in coordination chemistry, binding to metal centers in enzymes and altering their activity .
Cellular Effects
This compound affects various types of cells and cellular processes. It influences cell function by interacting with cell signaling pathways, gene expression, and cellular metabolism. The compound can modulate the activity of enzymes involved in these processes, leading to changes in cellular behavior. For example, it can affect the phosphorylation status of proteins, thereby influencing signal transduction pathways .
Molecular Mechanism
The molecular mechanism of action of this compound involves its binding interactions with biomolecules. It can act as an enzyme inhibitor or activator, depending on the context of its interaction. The compound can bind to the active sites of enzymes, altering their conformation and activity. Additionally, this compound can influence gene expression by interacting with transcription factors and other regulatory proteins .
Temporal Effects in Laboratory Settings
In laboratory settings, the effects of this compound change over time. The compound is relatively stable under standard conditions but can degrade upon exposure to air, forming its oxide. Long-term studies have shown that this compound can have lasting effects on cellular function, including sustained changes in enzyme activity and gene expression .
Dosage Effects in Animal Models
The effects of this compound vary with different dosages in animal models. At low doses, the compound can have beneficial effects on cellular processes, while at high doses, it may exhibit toxic or adverse effects. Threshold effects have been observed, where a certain concentration of the compound is required to elicit a significant biological response .
Metabolic Pathways
This compound is involved in various metabolic pathways. It interacts with enzymes and cofactors, influencing metabolic flux and metabolite levels. The compound can participate in redox reactions, acting as a reducing agent in certain biochemical processes. Its interactions with metabolic enzymes can lead to changes in the overall metabolic profile of cells .
Transport and Distribution
Within cells and tissues, this compound is transported and distributed through interactions with transporters and binding proteins. These interactions can affect the localization and accumulation of the compound, influencing its biological activity. The compound’s distribution within different cellular compartments can determine its specific effects on cellular function .
Subcellular Localization
This compound exhibits specific subcellular localization, which can affect its activity and function. Targeting signals and post-translational modifications direct the compound to particular compartments or organelles within the cell. This localization is crucial for its role in modulating cellular processes, as it ensures that the compound interacts with the appropriate biomolecules .
Preparation Methods
Tris(hydroxymethyl)phosphine is typically prepared by treating tetrakis(hydroxymethyl)phosphonium chloride with a strong base such as sodium hydroxide. The reaction can be represented as follows: [ \text{[P(CH₂OH)₄]Cl + NaOH → P(CH₂OH)₃ + H₂O + H₂C=O + NaCl} ] This compound can also be synthesized on a larger scale using triethylamine as both the base and the solvent .
Chemical Reactions Analysis
Tris(hydroxymethyl)phosphine undergoes various types of chemical reactions, including:
Oxidation: In the presence of air, it oxidizes to form the corresponding oxide.
Decomposition: Upon attempted distillation, it decomposes violently to phosphine and formaldehyde.
Complex Formation: It forms complexes with a variety of metals, which are soluble in water and methanol.
Conversion: When heated with hexamethylenetetramine, it converts to triazaphosphaadamantane
Scientific Research Applications
Tris(hydroxymethyl)phosphine has several applications in scientific research:
Medicine: Its reducing properties are utilized in medical research for the development of pharmaceuticals.
Industry: It is employed in the synthesis of flame-retardant materials and other industrial chemicals.
Comparison with Similar Compounds
Tris(hydroxymethyl)phosphine can be compared with other similar compounds such as:
- Tris(hydroxypropyl)phosphine
- Tris(2-carboxyethyl)phosphine
- Triphenylphosphine
- Tetrakis(hydroxymethyl)phosphonium chloride
What sets this compound apart is its multifunctionality, combining three alcohol groups with a tertiary phosphine, which makes it highly versatile in forming metal complexes and participating in various chemical reactions .
Properties
IUPAC Name |
bis(hydroxymethyl)phosphanylmethanol | |
---|---|---|
Source | PubChem | |
URL | https://pubchem.ncbi.nlm.nih.gov | |
Description | Data deposited in or computed by PubChem | |
InChI |
InChI=1S/C3H9O3P/c4-1-7(2-5)3-6/h4-6H,1-3H2 | |
Source | PubChem | |
URL | https://pubchem.ncbi.nlm.nih.gov | |
Description | Data deposited in or computed by PubChem | |
InChI Key |
JMXMXKRNIYCNRV-UHFFFAOYSA-N | |
Source | PubChem | |
URL | https://pubchem.ncbi.nlm.nih.gov | |
Description | Data deposited in or computed by PubChem | |
Canonical SMILES |
C(O)P(CO)CO | |
Source | PubChem | |
URL | https://pubchem.ncbi.nlm.nih.gov | |
Description | Data deposited in or computed by PubChem | |
Molecular Formula |
C3H9O3P | |
Source | PubChem | |
URL | https://pubchem.ncbi.nlm.nih.gov | |
Description | Data deposited in or computed by PubChem | |
DSSTOX Substance ID |
DTXSID0062634 | |
Record name | Methanol, phosphinidynetris- | |
Source | EPA DSSTox | |
URL | https://comptox.epa.gov/dashboard/DTXSID0062634 | |
Description | DSSTox provides a high quality public chemistry resource for supporting improved predictive toxicology. | |
Molecular Weight |
124.08 g/mol | |
Source | PubChem | |
URL | https://pubchem.ncbi.nlm.nih.gov | |
Description | Data deposited in or computed by PubChem | |
CAS No. |
2767-80-8 | |
Record name | Tris(hydroxymethyl)phosphine | |
Source | CAS Common Chemistry | |
URL | https://commonchemistry.cas.org/detail?cas_rn=2767-80-8 | |
Description | CAS Common Chemistry is an open community resource for accessing chemical information. Nearly 500,000 chemical substances from CAS REGISTRY cover areas of community interest, including common and frequently regulated chemicals, and those relevant to high school and undergraduate chemistry classes. This chemical information, curated by our expert scientists, is provided in alignment with our mission as a division of the American Chemical Society. | |
Explanation | The data from CAS Common Chemistry is provided under a CC-BY-NC 4.0 license, unless otherwise stated. | |
Record name | Tris(hydroxymethyl)phosphine | |
Source | ChemIDplus | |
URL | https://pubchem.ncbi.nlm.nih.gov/substance/?source=chemidplus&sourceid=0002767808 | |
Description | ChemIDplus is a free, web search system that provides access to the structure and nomenclature authority files used for the identification of chemical substances cited in National Library of Medicine (NLM) databases, including the TOXNET system. | |
Record name | Methanol, 1,1',1''-phosphinidynetris- | |
Source | EPA Chemicals under the TSCA | |
URL | https://www.epa.gov/chemicals-under-tsca | |
Description | EPA Chemicals under the Toxic Substances Control Act (TSCA) collection contains information on chemicals and their regulations under TSCA, including non-confidential content from the TSCA Chemical Substance Inventory and Chemical Data Reporting. | |
Record name | Methanol, phosphinidynetris- | |
Source | EPA DSSTox | |
URL | https://comptox.epa.gov/dashboard/DTXSID0062634 | |
Description | DSSTox provides a high quality public chemistry resource for supporting improved predictive toxicology. | |
Record name | Phosphinidynetrimethanol | |
Source | European Chemicals Agency (ECHA) | |
URL | https://echa.europa.eu/substance-information/-/substanceinfo/100.018.587 | |
Description | The European Chemicals Agency (ECHA) is an agency of the European Union which is the driving force among regulatory authorities in implementing the EU's groundbreaking chemicals legislation for the benefit of human health and the environment as well as for innovation and competitiveness. | |
Explanation | Use of the information, documents and data from the ECHA website is subject to the terms and conditions of this Legal Notice, and subject to other binding limitations provided for under applicable law, the information, documents and data made available on the ECHA website may be reproduced, distributed and/or used, totally or in part, for non-commercial purposes provided that ECHA is acknowledged as the source: "Source: European Chemicals Agency, http://echa.europa.eu/". Such acknowledgement must be included in each copy of the material. ECHA permits and encourages organisations and individuals to create links to the ECHA website under the following cumulative conditions: Links can only be made to webpages that provide a link to the Legal Notice page. | |
Record name | TRIS(HYDROXYMETHYL)PHOSPHINE | |
Source | FDA Global Substance Registration System (GSRS) | |
URL | https://gsrs.ncats.nih.gov/ginas/app/beta/substances/Y6TG7WF7OQ | |
Description | The FDA Global Substance Registration System (GSRS) enables the efficient and accurate exchange of information on what substances are in regulated products. Instead of relying on names, which vary across regulatory domains, countries, and regions, the GSRS knowledge base makes it possible for substances to be defined by standardized, scientific descriptions. | |
Explanation | Unless otherwise noted, the contents of the FDA website (www.fda.gov), both text and graphics, are not copyrighted. They are in the public domain and may be republished, reprinted and otherwise used freely by anyone without the need to obtain permission from FDA. Credit to the U.S. Food and Drug Administration as the source is appreciated but not required. | |
Synthesis routes and methods I
Procedure details
Synthesis routes and methods II
Procedure details
Synthesis routes and methods III
Procedure details
Retrosynthesis Analysis
AI-Powered Synthesis Planning: Our tool employs the Template_relevance Pistachio, Template_relevance Bkms_metabolic, Template_relevance Pistachio_ringbreaker, Template_relevance Reaxys, Template_relevance Reaxys_biocatalysis model, leveraging a vast database of chemical reactions to predict feasible synthetic routes.
One-Step Synthesis Focus: Specifically designed for one-step synthesis, it provides concise and direct routes for your target compounds, streamlining the synthesis process.
Accurate Predictions: Utilizing the extensive PISTACHIO, BKMS_METABOLIC, PISTACHIO_RINGBREAKER, REAXYS, REAXYS_BIOCATALYSIS database, our tool offers high-accuracy predictions, reflecting the latest in chemical research and data.
Strategy Settings
Precursor scoring | Relevance Heuristic |
---|---|
Min. plausibility | 0.01 |
Model | Template_relevance |
Template Set | Pistachio/Bkms_metabolic/Pistachio_ringbreaker/Reaxys/Reaxys_biocatalysis |
Top-N result to add to graph | 6 |
Feasible Synthetic Routes
Q1: What is the molecular formula and weight of Tris(hydroxymethyl)phosphine (THP)?
A1: The molecular formula of THP is C3H9O3P and its molecular weight is 124.08 g/mol.
Q2: What is unique about the 31P NMR chemical shift of THP and its derivatives?
A2: Dioxaphosphabicyclo[2.2.2]octanes, a class of THP derivatives, exhibit highly upfield 31P NMR chemical shifts ranging from -59 to -70 ppm. This characteristic is attributed to their caged structure. []
Q3: How does THP perform as a coupling agent for enzyme immobilization?
A3: THP effectively immobilizes enzymes like β-galactosidase onto chitosan. [, ] THP-immobilized β-galactosidase exhibits superior thermostability and storage stability compared to free or glutaraldehyde-immobilized enzymes. [] This makes THP a promising coupling agent for various biotechnological applications.
Q4: How stable are this compound (THP) complexes in water?
A5: Diorganoplatinum(II) complexes incorporating THP as a ligand show significant stability in water. [] This water solubility opens avenues for applications in aqueous-phase catalysis.
Q5: Can THP be used in biphasic catalysis?
A6: Yes, both nickel(0) and palladium(0) complexes with THP can catalyze buta-1,3-diene oligomerization or telomerization in aqueous biphasic systems. [] These reactions are particularly noteworthy as they proceed without the need for cosolvents or modifiers, highlighting the unique properties of THP as a ligand.
Q6: How does the structure of the attached rhodium carbonyl cluster on a THP-modified silica surface change with THP loading, and what is the impact on hydroformylation reactions?
A7: The number of THP ligands coordinating to Rh4 clusters on silica surfaces is dependent on THP loading. At higher loading (e.g., 6.2 wt% THP), Rh4 clusters are coordinated by two THP ligands and display structural distortion. Conversely, at lower loading (1.6 wt% THP), the clusters bind to one THP ligand and remain undistorted. Interestingly, the distorted Rh4 framework coordinated by two THP ligands exhibits significantly higher activity in hydroformylation reactions compared to monosubstituted clusters. []
Q7: What role does this compound (THP) play in parahydrogen induced polarization (PHIP)?
A8: THP serves as a monodentate phosphine ligand in a water-soluble Rh(I)-based catalyst, [Rh(I)(norbornadiene)(THP)2]+[BF4]-, used for PHIP. This catalyst demonstrates high efficiency in hydrogenation reactions, even in the presence of oxidized THP, and yields substantial 13C polarization for the parahydrogen addition product. []
Q8: Can this compound (THP) be used to synthesize nanoparticles?
A9: Yes, THP-capped gold nanoparticles demonstrate the ability to bind to DNA, even though both are negatively charged. These nanoparticles act as catalysts for electroless gold plating, enabling the fabrication of nanowires with potential applications in nanoelectronics. []
Q9: How is this compound (THP) used in the synthesis of amphiphilic Janus gold nanoparticles?
A10: THP, alongside triphenylphosphine and their oxides, acts as a stabilizing agent in the synthesis of ultrafine amphiphilic Janus gold nanoparticles via a toluene/water emulsion reaction. This method offers a high yielding (up to 93.3%) and scalable route for producing these unique nanoparticles, which have potential applications in various fields. [, ]
Q10: How does this compound (THP) contribute to the synthesis of phosphorus-nitrogen polymers used for flameproofing cellulose?
A12: Polymers synthesized from THP and ammonia demonstrate promising flame-retardant properties. These polymers exhibit comparable thermal degradation characteristics to those derived from tetrakis(hydroxymethyl)phosphonium chloride (THPC), although their chemical structures differ. This finding highlights the potential of THP as a building block for developing novel flame-retardant materials. []
Q11: How does the structure of this compound (THP) derivatives influence their catalytic activity in hydroformylation reactions?
A13: The steric bulk of substituents on dioxaphosphabicyclo[2.2.2]octanes, a class of THP derivatives, plays a significant role in rhodium-catalyzed hydroformylation of 1-octene. Increasing steric bulk around the coordination environment of the catalyst leads to a threefold enhancement in aldehyde yield, while maintaining consistent linear selectivity to n-nonanal (68%). []
Q12: How does the stability of this compound (THP) immobilized beta-galactosidase compare to free and glutaraldehyde-immobilized enzymes?
A14: THP-immobilized beta-galactosidase demonstrates superior thermostability and activity retention during storage compared to both free and glutaraldehyde-immobilized forms. Notably, it retains over 80% of its initial activity after 6 weeks of storage at 4°C. This enhanced stability makes THP immobilization a promising strategy for improving the shelf life and performance of enzyme-based products. []
Q13: How does this compound (THP) contribute to the stability of silver nanoparticle/multiwalled carbon nanotube hybrids used as electrocatalysts?
A15: THP, when used as a capping agent for silver nanoparticles, enhances the stability of silver nanoparticle/multiwalled carbon nanotube hybrids, making them highly efficient and durable electrocatalysts for oxygen reduction reactions in alkaline media. These hybrids demonstrate superior long-term stability and methanol tolerance compared to traditional platinum/carbon catalysts, highlighting their potential for fuel cell applications. []
Q14: Can this compound (THP) be used in the synthesis of biomaterials?
A17: Yes, THP facilitates the rapid crosslinking of modular proteins containing resilin sequences, enabling the creation of hydrogels suitable for tissue engineering applications. These hydrogels possess mechanical properties comparable to human cartilage, demonstrating their potential in regenerative medicine. []
Q15: What are the potential applications of this compound (THP) in drug delivery?
A15: While the provided research primarily focuses on other applications of THP, its ability to form stable complexes with various metals, particularly platinum, suggests its potential utility in drug delivery, especially for platinum-based anticancer drugs. Further research is needed to explore this avenue.
Q16: How does this compound (THP) impact the direct synthesis of hydrogen peroxide (H2O2) using platinum catalysts?
A19: While platinum exhibits high activity in activating hydrogen for H2O2 synthesis, it suffers from low selectivity. The addition of THP, unfortunately, does not improve the selectivity and instead, unselectively inhibits the H2-O2 reaction. This finding highlights the importance of careful additive selection for optimizing H2O2 production. [, ]
Q17: Can this compound (THP) be used as a cross-linking agent?
A20: Yes, THP can act as a cross-linking agent due to its three reactive hydroxyl groups. This property is particularly valuable in preparing hydrogels from resilin-based proteins, where THP facilitates the formation of materials with tunable mechanical properties for potential biomedical applications. []
Disclaimer and Information on In-Vitro Research Products
Please be aware that all articles and product information presented on BenchChem are intended solely for informational purposes. The products available for purchase on BenchChem are specifically designed for in-vitro studies, which are conducted outside of living organisms. In-vitro studies, derived from the Latin term "in glass," involve experiments performed in controlled laboratory settings using cells or tissues. It is important to note that these products are not categorized as medicines or drugs, and they have not received approval from the FDA for the prevention, treatment, or cure of any medical condition, ailment, or disease. We must emphasize that any form of bodily introduction of these products into humans or animals is strictly prohibited by law. It is essential to adhere to these guidelines to ensure compliance with legal and ethical standards in research and experimentation.