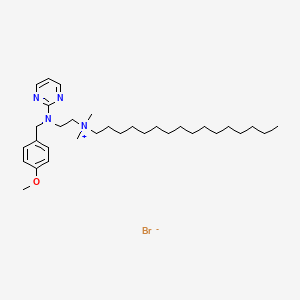
Thonzonium bromide
Overview
Description
Thonzonium bromide is a monocationic detergent and surfactant widely used in pharmaceutical formulations. It is known for its ability to promote tissue contact by dispersing and penetrating cellular debris and exudate. This property makes it particularly useful in ear and nasal drops to enhance the effectiveness of the active ingredients .
Mechanism of Action
Target of Action
Thonzonium bromide is a monocationic surface-active agent with surfactant and detergent properties . It is primarily targeted at cellular debris and exudate , enhancing their dispersion and penetration . This promotes tissue contact of the administered medication . This compound also inhibits vacuolar ATPase in pathogenic fungi such as Candida albicans .
Mode of Action
This compound acts as a surface-active agent in nasal and ear drops, potentiating and lengthening the time of skin contact with the active compounds and penetration through the cellular debris . It uncouples vacuolar ATPase in pathogenic fungi such as Candida albicans, inhibiting cell growth in vitro . It interacts with the tether of subunit a (Vph1p) of the membrane-bound V0 subunit that forms the proton transport path .
Biochemical Pathways
This compound affects the ERK1/2 and p38 pathways and causes mitochondrial uncoupling . It suppresses ERK 1/2 phosphorylation and increases BAX expression and p38 phosphorylation . It also affects Ca2+ homeostasis in both mitochondrial and cytosolic compartments .
Pharmacokinetics
It is known that it is used as a monocationic surfactant and detergent to help penetration of active ingredients through cellular debris for its antibacterial action .
Result of Action
This compound causes dispersion and penetration of cellular debris and exudate, thereby promoting tissue contact of the active ingredients contained in the administered medication . It inhibits cell growth in vitro by uncoupling vacuolar ATPase in pathogenic fungi such as Candida albicans . It also inhibits RANKL-induced osteoclast formation and bone resorption in vitro and prevents LPS-induced bone loss in vivo .
Action Environment
It is widely used as an additive to ear and nasal drops to enhance dispersion and penetration of cellular debris and exudate , suggesting that its efficacy may be influenced by the specific conditions of these environments.
Biochemical Analysis
Biochemical Properties
Thonzonium bromide plays a significant role in biochemical reactions due to its surface-active properties. It interacts with various enzymes, proteins, and other biomolecules. One of its primary interactions is with vacuolar ATPase (V-ATPase), where it acts as an inhibitor. This compound uncouples the V-ATPase function by blocking proton transport without inhibiting ATP hydrolysis . This interaction disrupts the proton gradient, affecting cellular pH and leading to cell death in pathogenic fungi such as Candida albicans .
Cellular Effects
This compound has notable effects on various cell types and cellular processes. It influences cell function by affecting cell signaling pathways, gene expression, and cellular metabolism. For instance, in malignant pleural mesothelioma cells, this compound inhibits the progression of the disease by regulating the ERK1/2 and p38 pathways and causing mitochondrial uncoupling . This compound also affects calcium homeostasis in both mitochondrial and cytosolic compartments, impacting mitochondrial function, respiration, and ATP production .
Molecular Mechanism
At the molecular level, this compound exerts its effects through several mechanisms. It uncouples vacuolar ATPase in pathogenic fungi, inhibiting cell growth by interacting with the tether of subunit a (Vph1p) of the membrane-bound V0 subunit that forms the proton transport path . Additionally, this compound inhibits the main proteases of human coronaviruses by occupying the catalytic site and inducing conformational changes in the protease . This broad-spectrum inhibitory activity highlights its potential as an antiviral agent.
Temporal Effects in Laboratory Settings
In laboratory settings, the effects of this compound change over time. Studies have shown that it remains stable and effective in promoting tissue contact and penetration of active ingredients through cellular debris . Long-term effects on cellular function have been observed, such as the inhibition of osteoclast formation and bone resorption in vitro and prevention of bone loss in vivo . These findings suggest that this compound maintains its bioactivity over extended periods.
Dosage Effects in Animal Models
The effects of this compound vary with different dosages in animal models. In a study on malignant pleural mesothelioma, this compound was administered at a dosage of 4 mg/kg intraperitoneally, twice a week for four weeks, resulting in increased overall survival compared to the control group . High doses of this compound may lead to toxic or adverse effects, emphasizing the importance of determining optimal dosages for therapeutic applications.
Metabolic Pathways
This compound is involved in several metabolic pathways, including the regulation of the mevalonate pathway. It affects calcium homeostasis, mitochondrial function, respiration, and ATP production . By influencing these metabolic processes, this compound can alter metabolic flux and metabolite levels, contributing to its therapeutic effects.
Transport and Distribution
This compound is transported and distributed within cells and tissues through interactions with transporters and binding proteins. It interacts with the tether of subunit a (Vph1p) of the membrane-bound V0 subunit, forming the proton transport path . This interaction disrupts the essential pump function, preventing the redistribution of cytosolic protons into the vacuolar lumen, leading to acidification of the cytosol and cell death .
Subcellular Localization
This compound’s subcellular localization plays a crucial role in its activity and function. It targets specific compartments or organelles, such as the vacuolar ATPase in pathogenic fungi . By uncoupling the V-ATPase function, this compound disrupts proton transport and affects cellular pH, leading to cell death . This targeting mechanism highlights its potential as an antifungal and antiviral agent.
Preparation Methods
Synthetic Routes and Reaction Conditions
Thonzonium bromide can be synthesized through a series of chemical reactions involving the alkylation of a tertiary amine with a long-chain alkyl halide. The reaction typically involves the use of solvents such as dimethyl sulfoxide (DMSO) and catalysts to facilitate the process. The final product is purified through crystallization or other separation techniques .
Industrial Production Methods
In industrial settings, this compound is produced using large-scale reactors where the reaction conditions are carefully controlled to ensure high yield and purity. The process involves the use of high-purity reagents and advanced purification methods to obtain the final product suitable for pharmaceutical applications .
Chemical Reactions Analysis
Types of Reactions
Thonzonium bromide undergoes various chemical reactions, including:
Oxidation: this compound can be oxidized under specific conditions, leading to the formation of oxidized derivatives.
Reduction: It can also undergo reduction reactions, although these are less common.
Substitution: This compound can participate in substitution reactions, where the bromide ion is replaced by other nucleophiles
Common Reagents and Conditions
Oxidation: Common oxidizing agents include hydrogen peroxide and potassium permanganate.
Reduction: Reducing agents such as sodium borohydride can be used.
Substitution: Nucleophiles like hydroxide ions or other halides are commonly used in substitution reactions
Major Products Formed
The major products formed from these reactions depend on the specific conditions and reagents used. For example, oxidation may lead to the formation of oxidized thonzonium derivatives, while substitution reactions can yield various substituted products .
Scientific Research Applications
Thonzonium bromide has a wide range of scientific research applications:
Chemistry: It is used as a surfactant and detergent in various chemical reactions and processes.
Biology: this compound is employed in biological research to enhance the penetration of active ingredients in cellular studies.
Medicine: It is a key component in ear and nasal drops, promoting the dispersion and penetration of cellular debris and exudate.
Industry: This compound is used in industrial applications where its surfactant properties are beneficial, such as in cleaning and formulation processes
Comparison with Similar Compounds
Similar Compounds
- Decamethonium bromide
- Cetalkonium chloride
- Domiphen bromide
- Farnesol (structurally similar antimicrobial agent)
Uniqueness
Thonzonium bromide is unique due to its specific surfactant and detergent properties, which make it highly effective in promoting tissue contact and enhancing the penetration of active ingredients. Its ability to inhibit vacuolar ATPase and its antifungal properties further distinguish it from other similar compounds .
Properties
IUPAC Name |
hexadecyl-[2-[(4-methoxyphenyl)methyl-pyrimidin-2-ylamino]ethyl]-dimethylazanium;bromide | |
---|---|---|
Source | PubChem | |
URL | https://pubchem.ncbi.nlm.nih.gov | |
Description | Data deposited in or computed by PubChem | |
InChI |
InChI=1S/C32H55N4O.BrH/c1-5-6-7-8-9-10-11-12-13-14-15-16-17-18-27-36(2,3)28-26-35(32-33-24-19-25-34-32)29-30-20-22-31(37-4)23-21-30;/h19-25H,5-18,26-29H2,1-4H3;1H/q+1;/p-1 | |
Source | PubChem | |
URL | https://pubchem.ncbi.nlm.nih.gov | |
Description | Data deposited in or computed by PubChem | |
InChI Key |
WBWDWFZTSDZAIG-UHFFFAOYSA-M | |
Source | PubChem | |
URL | https://pubchem.ncbi.nlm.nih.gov | |
Description | Data deposited in or computed by PubChem | |
Canonical SMILES |
CCCCCCCCCCCCCCCC[N+](C)(C)CCN(CC1=CC=C(C=C1)OC)C2=NC=CC=N2.[Br-] | |
Source | PubChem | |
URL | https://pubchem.ncbi.nlm.nih.gov | |
Description | Data deposited in or computed by PubChem | |
Molecular Formula |
C32H55BrN4O | |
Source | PubChem | |
URL | https://pubchem.ncbi.nlm.nih.gov | |
Description | Data deposited in or computed by PubChem | |
DSSTOX Substance ID |
DTXSID1045326 | |
Record name | Thonzonium bromide | |
Source | EPA DSSTox | |
URL | https://comptox.epa.gov/dashboard/DTXSID1045326 | |
Description | DSSTox provides a high quality public chemistry resource for supporting improved predictive toxicology. | |
Molecular Weight |
591.7 g/mol | |
Source | PubChem | |
URL | https://pubchem.ncbi.nlm.nih.gov | |
Description | Data deposited in or computed by PubChem | |
CAS No. |
553-08-2 | |
Record name | Thonzonium bromide | |
Source | CAS Common Chemistry | |
URL | https://commonchemistry.cas.org/detail?cas_rn=553-08-2 | |
Description | CAS Common Chemistry is an open community resource for accessing chemical information. Nearly 500,000 chemical substances from CAS REGISTRY cover areas of community interest, including common and frequently regulated chemicals, and those relevant to high school and undergraduate chemistry classes. This chemical information, curated by our expert scientists, is provided in alignment with our mission as a division of the American Chemical Society. | |
Explanation | The data from CAS Common Chemistry is provided under a CC-BY-NC 4.0 license, unless otherwise stated. | |
Record name | Thonzonium bromide [USAN:USP] | |
Source | ChemIDplus | |
URL | https://pubchem.ncbi.nlm.nih.gov/substance/?source=chemidplus&sourceid=0000553082 | |
Description | ChemIDplus is a free, web search system that provides access to the structure and nomenclature authority files used for the identification of chemical substances cited in National Library of Medicine (NLM) databases, including the TOXNET system. | |
Record name | THONZONIUM BROMIDE | |
Source | DTP/NCI | |
URL | https://dtp.cancer.gov/dtpstandard/servlet/dwindex?searchtype=NSC&outputformat=html&searchlist=760359 | |
Description | The NCI Development Therapeutics Program (DTP) provides services and resources to the academic and private-sector research communities worldwide to facilitate the discovery and development of new cancer therapeutic agents. | |
Explanation | Unless otherwise indicated, all text within NCI products is free of copyright and may be reused without our permission. Credit the National Cancer Institute as the source. | |
Record name | THONZONIUM BROMIDE | |
Source | DTP/NCI | |
URL | https://dtp.cancer.gov/dtpstandard/servlet/dwindex?searchtype=NSC&outputformat=html&searchlist=5648 | |
Description | The NCI Development Therapeutics Program (DTP) provides services and resources to the academic and private-sector research communities worldwide to facilitate the discovery and development of new cancer therapeutic agents. | |
Explanation | Unless otherwise indicated, all text within NCI products is free of copyright and may be reused without our permission. Credit the National Cancer Institute as the source. | |
Record name | Thonzonium bromide | |
Source | EPA DSSTox | |
URL | https://comptox.epa.gov/dashboard/DTXSID1045326 | |
Description | DSSTox provides a high quality public chemistry resource for supporting improved predictive toxicology. | |
Record name | Tonzonium bromide | |
Source | European Chemicals Agency (ECHA) | |
URL | https://echa.europa.eu/substance-information/-/substanceinfo/100.008.212 | |
Description | The European Chemicals Agency (ECHA) is an agency of the European Union which is the driving force among regulatory authorities in implementing the EU's groundbreaking chemicals legislation for the benefit of human health and the environment as well as for innovation and competitiveness. | |
Explanation | Use of the information, documents and data from the ECHA website is subject to the terms and conditions of this Legal Notice, and subject to other binding limitations provided for under applicable law, the information, documents and data made available on the ECHA website may be reproduced, distributed and/or used, totally or in part, for non-commercial purposes provided that ECHA is acknowledged as the source: "Source: European Chemicals Agency, http://echa.europa.eu/". Such acknowledgement must be included in each copy of the material. ECHA permits and encourages organisations and individuals to create links to the ECHA website under the following cumulative conditions: Links can only be made to webpages that provide a link to the Legal Notice page. | |
Record name | THONZONIUM BROMIDE | |
Source | FDA Global Substance Registration System (GSRS) | |
URL | https://gsrs.ncats.nih.gov/ginas/app/beta/substances/JI2B19CR0R | |
Description | The FDA Global Substance Registration System (GSRS) enables the efficient and accurate exchange of information on what substances are in regulated products. Instead of relying on names, which vary across regulatory domains, countries, and regions, the GSRS knowledge base makes it possible for substances to be defined by standardized, scientific descriptions. | |
Explanation | Unless otherwise noted, the contents of the FDA website (www.fda.gov), both text and graphics, are not copyrighted. They are in the public domain and may be republished, reprinted and otherwise used freely by anyone without the need to obtain permission from FDA. Credit to the U.S. Food and Drug Administration as the source is appreciated but not required. | |
Retrosynthesis Analysis
AI-Powered Synthesis Planning: Our tool employs the Template_relevance Pistachio, Template_relevance Bkms_metabolic, Template_relevance Pistachio_ringbreaker, Template_relevance Reaxys, Template_relevance Reaxys_biocatalysis model, leveraging a vast database of chemical reactions to predict feasible synthetic routes.
One-Step Synthesis Focus: Specifically designed for one-step synthesis, it provides concise and direct routes for your target compounds, streamlining the synthesis process.
Accurate Predictions: Utilizing the extensive PISTACHIO, BKMS_METABOLIC, PISTACHIO_RINGBREAKER, REAXYS, REAXYS_BIOCATALYSIS database, our tool offers high-accuracy predictions, reflecting the latest in chemical research and data.
Strategy Settings
Precursor scoring | Relevance Heuristic |
---|---|
Min. plausibility | 0.01 |
Model | Template_relevance |
Template Set | Pistachio/Bkms_metabolic/Pistachio_ringbreaker/Reaxys/Reaxys_biocatalysis |
Top-N result to add to graph | 6 |
Feasible Synthetic Routes
A: Thonzonium bromide exerts its effects through multiple mechanisms. Primarily, it acts as a cationic surfactant, disrupting bacterial cell membranes and causing leakage of cellular contents. [] Additionally, research indicates that it can uncouple Vacuolar ATPases (V-ATPases), inhibiting proton transport and disrupting pH homeostasis in cells. [, ]
ANone: this compound is a quaternary ammonium compound. While the provided research papers do not explicitly state its molecular formula and weight, these can be readily found in chemical databases. Unfortunately, the papers do not provide detailed spectroscopic data.
A: this compound's surfactant nature makes it an effective mucolytic agent, enhancing penetration of formulations in applications like nasal sprays. [, ] Additionally, it is incorporated into otic solutions to aid in the dispersion and penetration of other active ingredients. []
ANone: The provided research papers do not contain information regarding the use of computational chemistry, simulations, or QSAR models for this compound.
A: While specific Structure-Activity Relationship (SAR) studies for this compound are not detailed in the provided papers, research suggests that its long alkyl chain structure contributes to its ability to disrupt bacterial cell membranes. [] Furthermore, a study focusing on related quaternary ammonium compounds highlights the importance of aromatic functionality and its influence on interactions with copper surfaces during electroplating. []
A: The research papers primarily discuss this compound in the context of existing formulations, such as otic solutions [] and nasal sprays. [, ] One study investigates its thermal decomposition, but specific details are not provided. []
A: Yes, research indicates that this compound inhibits RANKL-induced osteoclast formation and bone resorption in vitro and has shown potential in preventing LPS-induced bone loss in vivo. []
A: Yes, recent research is investigating the impact of this compound on both oral and gut microbiomes. []
A: One research paper explores the use of pH-responsive polymer nanoparticles as carriers for this compound and structurally related drugs for enhanced anti-biofilm activity. [] This research highlights the potential for developing targeted therapies, particularly in the context of oral diseases.
Disclaimer and Information on In-Vitro Research Products
Please be aware that all articles and product information presented on BenchChem are intended solely for informational purposes. The products available for purchase on BenchChem are specifically designed for in-vitro studies, which are conducted outside of living organisms. In-vitro studies, derived from the Latin term "in glass," involve experiments performed in controlled laboratory settings using cells or tissues. It is important to note that these products are not categorized as medicines or drugs, and they have not received approval from the FDA for the prevention, treatment, or cure of any medical condition, ailment, or disease. We must emphasize that any form of bodily introduction of these products into humans or animals is strictly prohibited by law. It is essential to adhere to these guidelines to ensure compliance with legal and ethical standards in research and experimentation.