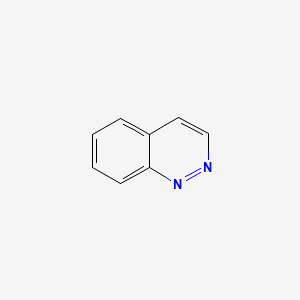
Cinnoline
Overview
Description
Cinnoline is an organic compound classified as a nitrogen-containing heterocycle. It is a bicyclic structure consisting of a benzene ring fused with a pyridazine ring, resulting in the molecular formula C₈H₆N₂.
Synthetic Routes and Reaction Conditions:
Microwave-Assisted Synthesis: One efficient method involves the reaction of 4-alkylpyridazine with nitrostyrene in dioxane/piperidine at 100°C using microwave irradiation.
PPA Catalyst Method: Another pathway utilizes polyphosphoric acid (PPA) as a catalyst at temperatures ranging from 100°C to 120°C.
Industrial Production Methods:
- Industrial production methods for this compound typically involve large-scale synthesis using optimized reaction conditions to ensure high yield and purity. Specific details on industrial methods are often proprietary and vary between manufacturers.
Types of Reactions:
Oxidation: this compound can undergo oxidation reactions, often resulting in the formation of various oxidized derivatives.
Reduction: Reduction reactions can convert this compound into its reduced forms, such as dihydrothis compound.
Substitution: this compound readily participates in substitution reactions, where functional groups can be introduced at different positions on the ring.
Common Reagents and Conditions:
Oxidation: Common oxidizing agents include potassium permanganate and chromium trioxide.
Reduction: Reducing agents such as sodium borohydride and lithium aluminum hydride are frequently used.
Substitution: Halogenation, nitration, and sulfonation reactions are common, often using reagents like chlorine, nitric acid, and sulfuric acid.
Major Products:
Mechanism of Action
Target of Action
Cinnoline is a significant pharmacological agent with a broad range of biological properties . It has been found to be a strong reversible BTK inhibitor , indicating that Bruton’s tyrosine kinase (BTK) could be a primary target of this compound. BTK plays a crucial role in the B cell receptor signaling pathway, which is essential for B cell development, differentiation, and signaling.
Pharmacokinetics
The development of this compound-based molecules has been noted to contribute significantly to the identification of lead compounds with optimized pharmacodynamic and pharmacokinetic properties
Result of Action
This compound derivatives exhibit a broad spectrum of pharmacological activities such as antibacterial, antifungal, antimalarial, anti-inflammatory, analgesic, anxiolytic, and antitumor activities . The exact molecular and cellular effects of this compound’s action would depend on the specific derivative and its targets.
Biochemical Analysis
Biochemical Properties
Cinnoline plays a crucial role in various biochemical reactions. It interacts with several enzymes, proteins, and other biomolecules, influencing their activity and function. For instance, this compound derivatives have been shown to inhibit certain enzymes such as tyrosine kinases, which are involved in cell signaling pathways. Additionally, this compound can bind to DNA and RNA, affecting gene expression and protein synthesis .
Cellular Effects
This compound exerts significant effects on various types of cells and cellular processes. It has been observed to influence cell function by modulating cell signaling pathways, gene expression, and cellular metabolism. For example, this compound derivatives have demonstrated anti-inflammatory and antitumor activities by inhibiting the proliferation of cancer cells and inducing apoptosis. Furthermore, this compound can affect the expression of genes involved in cell cycle regulation and apoptosis .
Molecular Mechanism
The molecular mechanism of this compound involves its interaction with biomolecules at the molecular level. This compound can bind to specific proteins and enzymes, leading to their inhibition or activation. For instance, this compound derivatives have been found to inhibit the activity of tyrosine kinases, which play a critical role in cell signaling and proliferation. Additionally, this compound can interact with DNA and RNA, leading to changes in gene expression and protein synthesis .
Temporal Effects in Laboratory Settings
In laboratory settings, the effects of this compound have been studied over time to understand its stability, degradation, and long-term effects on cellular function. This compound has been found to be relatively stable under physiological conditions, but its degradation products can also exhibit biological activity. Long-term studies have shown that this compound can have sustained effects on cellular function, including prolonged inhibition of cell proliferation and induction of apoptosis .
Dosage Effects in Animal Models
The effects of this compound vary with different dosages in animal models. At lower doses, this compound has been shown to exhibit therapeutic effects such as anti-inflammatory and antitumor activities. At higher doses, this compound can cause toxic or adverse effects, including hepatotoxicity and nephrotoxicity. Threshold effects have been observed, where the therapeutic effects of this compound are maximized at specific dosage ranges .
Metabolic Pathways
This compound is involved in various metabolic pathways, interacting with enzymes and cofactors that facilitate its metabolism. For example, this compound can be metabolized by cytochrome P450 enzymes in the liver, leading to the formation of active or inactive metabolites. These metabolites can further influence metabolic flux and alter the levels of other metabolites in the body .
Transport and Distribution
This compound is transported and distributed within cells and tissues through specific transporters and binding proteins. It can accumulate in certain tissues, such as the liver and kidneys, where it exerts its biological effects. The transport and distribution of this compound can also be influenced by its chemical properties, such as lipophilicity and molecular size .
Subcellular Localization
The subcellular localization of this compound is critical for its activity and function. This compound can be directed to specific compartments or organelles within the cell through targeting signals or post-translational modifications. For instance, this compound derivatives have been found to localize in the nucleus, where they can interact with DNA and RNA to modulate gene expression. Additionally, this compound can be targeted to the mitochondria, affecting cellular metabolism and energy production .
Scientific Research Applications
Cinnoline and its derivatives have a wide range of applications in scientific research:
Chemistry: Used as intermediates in the synthesis of complex organic molecules.
Biology: Studied for their interactions with biological macromolecules and potential as biochemical probes.
Medicine: this compound derivatives exhibit antibacterial, antifungal, antimalarial, anti-inflammatory, analgesic, anxiolytic, and antitumor activities.
Industry: Utilized in the development of pharmaceuticals, agrochemicals, and materials science.
Comparison with Similar Compounds
Quinoline: A heterocyclic aromatic organic compound with a nitrogen atom at position 1.
Isoquinoline: An isomer of quinoline with the nitrogen atom at position 2.
Phthalazine: A bicyclic compound with nitrogen atoms at positions 1 and 2, similar to cinnoline but with different chemical properties.
This compound’s unique structure and versatile reactivity make it a valuable compound in various fields of research and industry.
Properties
IUPAC Name |
cinnoline | |
---|---|---|
Source | PubChem | |
URL | https://pubchem.ncbi.nlm.nih.gov | |
Description | Data deposited in or computed by PubChem | |
InChI |
InChI=1S/C8H6N2/c1-2-4-8-7(3-1)5-6-9-10-8/h1-6H | |
Source | PubChem | |
URL | https://pubchem.ncbi.nlm.nih.gov | |
Description | Data deposited in or computed by PubChem | |
InChI Key |
WCZVZNOTHYJIEI-UHFFFAOYSA-N | |
Source | PubChem | |
URL | https://pubchem.ncbi.nlm.nih.gov | |
Description | Data deposited in or computed by PubChem | |
Canonical SMILES |
C1=CC=C2C(=C1)C=CN=N2 | |
Source | PubChem | |
URL | https://pubchem.ncbi.nlm.nih.gov | |
Description | Data deposited in or computed by PubChem | |
Molecular Formula |
C8H6N2 | |
Source | PubChem | |
URL | https://pubchem.ncbi.nlm.nih.gov | |
Description | Data deposited in or computed by PubChem | |
DSSTOX Substance ID |
DTXSID60179943 | |
Record name | Cinnoline | |
Source | EPA DSSTox | |
URL | https://comptox.epa.gov/dashboard/DTXSID60179943 | |
Description | DSSTox provides a high quality public chemistry resource for supporting improved predictive toxicology. | |
Molecular Weight |
130.15 g/mol | |
Source | PubChem | |
URL | https://pubchem.ncbi.nlm.nih.gov | |
Description | Data deposited in or computed by PubChem | |
CAS No. |
253-66-7 | |
Record name | Cinnoline | |
Source | CAS Common Chemistry | |
URL | https://commonchemistry.cas.org/detail?cas_rn=253-66-7 | |
Description | CAS Common Chemistry is an open community resource for accessing chemical information. Nearly 500,000 chemical substances from CAS REGISTRY cover areas of community interest, including common and frequently regulated chemicals, and those relevant to high school and undergraduate chemistry classes. This chemical information, curated by our expert scientists, is provided in alignment with our mission as a division of the American Chemical Society. | |
Explanation | The data from CAS Common Chemistry is provided under a CC-BY-NC 4.0 license, unless otherwise stated. | |
Record name | Cinnoline | |
Source | ChemIDplus | |
URL | https://pubchem.ncbi.nlm.nih.gov/substance/?source=chemidplus&sourceid=0000253667 | |
Description | ChemIDplus is a free, web search system that provides access to the structure and nomenclature authority files used for the identification of chemical substances cited in National Library of Medicine (NLM) databases, including the TOXNET system. | |
Record name | CINNOLINE | |
Source | DTP/NCI | |
URL | https://dtp.cancer.gov/dtpstandard/servlet/dwindex?searchtype=NSC&outputformat=html&searchlist=58374 | |
Description | The NCI Development Therapeutics Program (DTP) provides services and resources to the academic and private-sector research communities worldwide to facilitate the discovery and development of new cancer therapeutic agents. | |
Explanation | Unless otherwise indicated, all text within NCI products is free of copyright and may be reused without our permission. Credit the National Cancer Institute as the source. | |
Record name | Cinnoline | |
Source | EPA DSSTox | |
URL | https://comptox.epa.gov/dashboard/DTXSID60179943 | |
Description | DSSTox provides a high quality public chemistry resource for supporting improved predictive toxicology. | |
Record name | Cinnoline | |
Source | European Chemicals Agency (ECHA) | |
URL | https://echa.europa.eu/substance-information/-/substanceinfo/100.005.423 | |
Description | The European Chemicals Agency (ECHA) is an agency of the European Union which is the driving force among regulatory authorities in implementing the EU's groundbreaking chemicals legislation for the benefit of human health and the environment as well as for innovation and competitiveness. | |
Explanation | Use of the information, documents and data from the ECHA website is subject to the terms and conditions of this Legal Notice, and subject to other binding limitations provided for under applicable law, the information, documents and data made available on the ECHA website may be reproduced, distributed and/or used, totally or in part, for non-commercial purposes provided that ECHA is acknowledged as the source: "Source: European Chemicals Agency, http://echa.europa.eu/". Such acknowledgement must be included in each copy of the material. ECHA permits and encourages organisations and individuals to create links to the ECHA website under the following cumulative conditions: Links can only be made to webpages that provide a link to the Legal Notice page. | |
Record name | CINNOLINE | |
Source | FDA Global Substance Registration System (GSRS) | |
URL | https://gsrs.ncats.nih.gov/ginas/app/beta/substances/N5KD6I506O | |
Description | The FDA Global Substance Registration System (GSRS) enables the efficient and accurate exchange of information on what substances are in regulated products. Instead of relying on names, which vary across regulatory domains, countries, and regions, the GSRS knowledge base makes it possible for substances to be defined by standardized, scientific descriptions. | |
Explanation | Unless otherwise noted, the contents of the FDA website (www.fda.gov), both text and graphics, are not copyrighted. They are in the public domain and may be republished, reprinted and otherwise used freely by anyone without the need to obtain permission from FDA. Credit to the U.S. Food and Drug Administration as the source is appreciated but not required. | |
Synthesis routes and methods I
Procedure details
Synthesis routes and methods II
Procedure details
Retrosynthesis Analysis
AI-Powered Synthesis Planning: Our tool employs the Template_relevance Pistachio, Template_relevance Bkms_metabolic, Template_relevance Pistachio_ringbreaker, Template_relevance Reaxys, Template_relevance Reaxys_biocatalysis model, leveraging a vast database of chemical reactions to predict feasible synthetic routes.
One-Step Synthesis Focus: Specifically designed for one-step synthesis, it provides concise and direct routes for your target compounds, streamlining the synthesis process.
Accurate Predictions: Utilizing the extensive PISTACHIO, BKMS_METABOLIC, PISTACHIO_RINGBREAKER, REAXYS, REAXYS_BIOCATALYSIS database, our tool offers high-accuracy predictions, reflecting the latest in chemical research and data.
Strategy Settings
Precursor scoring | Relevance Heuristic |
---|---|
Min. plausibility | 0.01 |
Model | Template_relevance |
Template Set | Pistachio/Bkms_metabolic/Pistachio_ringbreaker/Reaxys/Reaxys_biocatalysis |
Top-N result to add to graph | 6 |
Feasible Synthetic Routes
Q1: What is Cinnoline?
A1: this compound, also known as 1,2-benzodiazine or benzopyridazine, is a heterocyclic aromatic compound with the molecular formula C8H6N2. It features a six-membered ring containing two nitrogen atoms, making it a diazanaphthalene. This compound derivatives have garnered significant interest in synthetic and medicinal chemistry due to their diverse pharmacological activities. [, , , , , , , , , , , , , , , , , , , , , , , , , , , ]
Q2: Can you provide some historical context on this compound research?
A2: this compound was first synthesized in 1883 by Von Richter, marking a milestone in heterocyclic chemistry. Since then, researchers have dedicated significant effort to developing novel synthetic routes and exploring the diverse biological activities of this compound derivatives. Early research focused on fundamental chemistry and reactivity, while more recent studies emphasize pharmacological applications and structure-activity relationships. [, , ]
Q3: What spectroscopic data are typically used to characterize cinnolines?
A3: Cinnolines and their derivatives are commonly characterized using a combination of spectroscopic techniques:
- NMR Spectroscopy: ¹H NMR and 13C NMR spectroscopy provide valuable information about the structure and substitution patterns in this compound derivatives. For example, the 1H NMR spectra of cinnolines generally show a characteristic deshielding order for the protons: 3-proton > 8-proton > 4-proton. [, ]
- UV-Vis Spectroscopy: This technique is useful for analyzing the electronic structure and conjugation within this compound derivatives, revealing insights into their photophysical properties. [, , ]
- Mass Spectrometry: This method helps determine the molecular weight and fragmentation patterns of cinnolines, aiding in structural elucidation. []
- IR Spectroscopy: This technique can identify functional groups present in this compound derivatives, such as carbonyl groups in cinnolones. [, ]
Q4: How does the presence of methoxy-groups influence the reactivity of cinnolines?
A6: Methoxy substituents can significantly impact the reactivity of cinnolines, particularly in methylation and quaternization reactions. For example, 3-methoxy-4(1H)-cinnolone selectively undergoes methylation at N(1) to form 3-methoxy-1-methyl-4-cinnolone. [] In contrast, 5-, 6-, 7-, and 8-methoxy-4(1H)-cinnolones predominantly yield the 2-methylcinnolinium-4-olates, with minor amounts of the 1-methyl-4-cinnolone isomers. [] Similar trends are observed in quaternization reactions with methyl iodide, where the position of the methoxy group dictates the major product. [] These findings underscore the importance of considering electronic and steric effects of substituents when designing synthetic routes for specific this compound derivatives.
Q5: Can you describe the fragmentation patterns of cinnolines observed in mass spectrometry?
A5: Mass spectrometry studies on cinnolines reveal distinct fragmentation patterns depending on the nature and position of substituents:
- Unsubstituted this compound: The fragmentation of this compound (C8H6N2) proceeds through consecutive losses of N2 and C2H2. []
- 4-Styrylthis compound and 4,4′-Dicinnolyl: Fragmentation of these compounds leads to the formation of characteristic ions, including the "dibenzoladderene" ion (from 4,4′-dicinnolyl) and the 1-phenylbenzodibutylene ion (from 4-styrylthis compound). []
- 4-Styrylquinoline: This compound exhibits an interesting fragmentation pathway involving hydrogen loss during ring rearrangement, resulting in the highly resonance-stabilized 3-styrylbenzocyclopentadieneoneimine ion, which subsequently loses nitrogen. []
- Substituted Cinnolines: Other substituted cinnolines display diverse fragmentation pathways. For instance, 4-methylthis compound fragments through a "1,2-diazatropylium" ion, while nitrocinnolines initially lose their nitro substituent. Aminocinnolines, on the other hand, typically lose N2 first, and 4-cinnolone undergoes consecutive losses of two HCN units and CO. []
Q6: What types of reactions are commonly utilized to functionalize the this compound core?
A6: this compound derivatives can be further functionalized using a variety of reactions to install desired substituents and modify their properties:
- Nitration: this compound and its derivatives can undergo nitration reactions, with the position of nitration being influenced by factors such as the reaction temperature, concentration of sulfuric acid, and the presence of N-oxides. []
- Nucleophilic Aromatic Substitution: Halogenated cinnolines, particularly those with halogens at the 4-position, can undergo nucleophilic aromatic substitution reactions with various nucleophiles, such as amines, alkoxides, and thiols. [, ] This provides a versatile approach for introducing a wide range of substituents onto the this compound core.
- Condensation Reactions: this compound derivatives containing amino or carbonyl groups can participate in condensation reactions to form fused heterocyclic systems. For example, reactions with malononitrile, ethyl cyanoacetate, or hydrazine can lead to the formation of pyrido[3,2-c]cinnolines, pyrimidino[5,4-c]cinnolines, or pyrazolo[4,3-c]cinnolines, respectively. []
Disclaimer and Information on In-Vitro Research Products
Please be aware that all articles and product information presented on BenchChem are intended solely for informational purposes. The products available for purchase on BenchChem are specifically designed for in-vitro studies, which are conducted outside of living organisms. In-vitro studies, derived from the Latin term "in glass," involve experiments performed in controlled laboratory settings using cells or tissues. It is important to note that these products are not categorized as medicines or drugs, and they have not received approval from the FDA for the prevention, treatment, or cure of any medical condition, ailment, or disease. We must emphasize that any form of bodily introduction of these products into humans or animals is strictly prohibited by law. It is essential to adhere to these guidelines to ensure compliance with legal and ethical standards in research and experimentation.