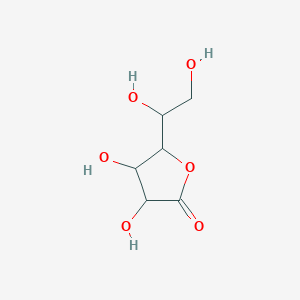
D-Galactonic acid, gamma-lactone
Overview
Description
“D-Galactonic acid, gamma-lactone” is a chemical compound with the formula C6H10O6 and a molecular weight of 178.1400 . It is also known by other names such as γ-D-Galactonolactone, D-Galactono-γ-lactone, D-Galactono-1,4-lactone, Galactonic acid, γ-lactone, D-, and 1,4-D-Galactonolactone .
Molecular Structure Analysis
The molecular structure of “D-Galactonic acid, gamma-lactone” can be represented as a 2D Mol file or a computed 3D SD file . The 3D structure provides a more detailed view of the spatial arrangement of atoms and the chemical bonds that hold the atoms together .
Scientific Research Applications
Biochemical Analysis
D-Galactonic acid gamma-lactone has been utilized in biochemical analysis, particularly in urine analysis. A study developed a stable isotope dilution assay for the sensitive determination of D-galactonic acid, highlighting its importance in studying the variability of D-galactonate excretion in healthy subjects and galactosemic patients (Schadewaldt et al., 2004).
Microbial Metabolism
In microbial metabolism, D-galactonic acid gamma-lactone plays a significant role. Research on Aspergillus niger demonstrated the fungus's ability to utilize D-galactose as a carbon source, implicating the metabolism of D-galactonic acid gamma-lactone in the process. The study detailed how D-galactose was converted to D-galactonic acid-gamma-lactone, suggesting a non-phosphorylated route of galactose breakdown in the cell-free extracts of Aspergillus niger (Elshafei & Abdel-Fatah, 2001).
Enzymatic Functions and Ascorbic Acid Biosynthesis
The enzymatic functions of D-galactonic acid gamma-lactone have been explored, particularly in the biosynthesis of L-ascorbic acid (vitamin C). SMP30, identified as gluconolactonase, was found to hydrolyze various aldonolactones, including D-galactonic acid gamma-lactone. This enzyme's absence in knockout mice led to symptoms of scurvy, underscoring its crucial role in L-ascorbic acid biosynthesis (Kondo et al., 2006). In another study, the synthesis of ascorbic acid in plant tissues was linked to the mitochondrial electron transport chain, involving galactono-gamma-lactone dehydrogenase (GLDH) and emphasizing the pivotal role of D-galactonic acid gamma-lactone in this process (Bartoli et al., 2000).
Chemical Synthesis and Applications
D-Galactonic acid gamma-lactone has also been recognized for its potential in chemical synthesis. Its derivative, D-galactono-1,4-lactone, was utilized as a chiral starting material for synthesizing various molecules, showcasing its versatility in chemical synthesis (Rivas et al., 2018). Moreover, derivatives of D- and L-galactonic acids have been synthesized as precursors for stereoregular polymers, highlighting the utility of D-galactonic acid gamma-lactone in polymer science (Zaliz & Varela, 2003).
Safety And Hazards
properties
IUPAC Name |
5-(1,2-dihydroxyethyl)-3,4-dihydroxyoxolan-2-one | |
---|---|---|
Source | PubChem | |
URL | https://pubchem.ncbi.nlm.nih.gov | |
Description | Data deposited in or computed by PubChem | |
InChI |
InChI=1S/C6H10O6/c7-1-2(8)5-3(9)4(10)6(11)12-5/h2-5,7-10H,1H2 | |
Source | PubChem | |
URL | https://pubchem.ncbi.nlm.nih.gov | |
Description | Data deposited in or computed by PubChem | |
InChI Key |
SXZYCXMUPBBULW-UHFFFAOYSA-N | |
Source | PubChem | |
URL | https://pubchem.ncbi.nlm.nih.gov | |
Description | Data deposited in or computed by PubChem | |
Canonical SMILES |
C(C(C1C(C(C(=O)O1)O)O)O)O | |
Source | PubChem | |
URL | https://pubchem.ncbi.nlm.nih.gov | |
Description | Data deposited in or computed by PubChem | |
Molecular Formula |
C6H10O6 | |
Source | PubChem | |
URL | https://pubchem.ncbi.nlm.nih.gov | |
Description | Data deposited in or computed by PubChem | |
Molecular Weight |
178.14 g/mol | |
Source | PubChem | |
URL | https://pubchem.ncbi.nlm.nih.gov | |
Description | Data deposited in or computed by PubChem | |
Product Name |
D-Galactonic acid, gamma-lactone | |
CAS RN |
2782-07-2, 6322-07-2, 1668-08-2, 26301-79-1 | |
Record name | D-galactono-1,4-lactone | |
Source | DTP/NCI | |
URL | https://dtp.cancer.gov/dtpstandard/servlet/dwindex?searchtype=NSC&outputformat=html&searchlist=102762 | |
Description | The NCI Development Therapeutics Program (DTP) provides services and resources to the academic and private-sector research communities worldwide to facilitate the discovery and development of new cancer therapeutic agents. | |
Explanation | Unless otherwise indicated, all text within NCI products is free of copyright and may be reused without our permission. Credit the National Cancer Institute as the source. | |
Record name | NSC34392 | |
Source | DTP/NCI | |
URL | https://dtp.cancer.gov/dtpstandard/servlet/dwindex?searchtype=NSC&outputformat=html&searchlist=34392 | |
Description | The NCI Development Therapeutics Program (DTP) provides services and resources to the academic and private-sector research communities worldwide to facilitate the discovery and development of new cancer therapeutic agents. | |
Explanation | Unless otherwise indicated, all text within NCI products is free of copyright and may be reused without our permission. Credit the National Cancer Institute as the source. | |
Record name | NSC25282 | |
Source | DTP/NCI | |
URL | https://dtp.cancer.gov/dtpstandard/servlet/dwindex?searchtype=NSC&outputformat=html&searchlist=25282 | |
Description | The NCI Development Therapeutics Program (DTP) provides services and resources to the academic and private-sector research communities worldwide to facilitate the discovery and development of new cancer therapeutic agents. | |
Explanation | Unless otherwise indicated, all text within NCI products is free of copyright and may be reused without our permission. Credit the National Cancer Institute as the source. | |
Record name | Mannonic acid, D- | |
Source | DTP/NCI | |
URL | https://dtp.cancer.gov/dtpstandard/servlet/dwindex?searchtype=NSC&outputformat=html&searchlist=1971 | |
Description | The NCI Development Therapeutics Program (DTP) provides services and resources to the academic and private-sector research communities worldwide to facilitate the discovery and development of new cancer therapeutic agents. | |
Explanation | Unless otherwise indicated, all text within NCI products is free of copyright and may be reused without our permission. Credit the National Cancer Institute as the source. | |
Record name | D-Galactonic acid, .gamma.-lactone | |
Source | EPA Chemicals under the TSCA | |
URL | https://www.epa.gov/chemicals-under-tsca | |
Description | EPA Chemicals under the Toxic Substances Control Act (TSCA) collection contains information on chemicals and their regulations under TSCA, including non-confidential content from the TSCA Chemical Substance Inventory and Chemical Data Reporting. | |
Synthesis routes and methods
Procedure details
Retrosynthesis Analysis
AI-Powered Synthesis Planning: Our tool employs the Template_relevance Pistachio, Template_relevance Bkms_metabolic, Template_relevance Pistachio_ringbreaker, Template_relevance Reaxys, Template_relevance Reaxys_biocatalysis model, leveraging a vast database of chemical reactions to predict feasible synthetic routes.
One-Step Synthesis Focus: Specifically designed for one-step synthesis, it provides concise and direct routes for your target compounds, streamlining the synthesis process.
Accurate Predictions: Utilizing the extensive PISTACHIO, BKMS_METABOLIC, PISTACHIO_RINGBREAKER, REAXYS, REAXYS_BIOCATALYSIS database, our tool offers high-accuracy predictions, reflecting the latest in chemical research and data.
Strategy Settings
Precursor scoring | Relevance Heuristic |
---|---|
Min. plausibility | 0.01 |
Model | Template_relevance |
Template Set | Pistachio/Bkms_metabolic/Pistachio_ringbreaker/Reaxys/Reaxys_biocatalysis |
Top-N result to add to graph | 6 |
Feasible Synthetic Routes
Q & A
Q1: What is the mechanism of action of D-talono-1,4-lactone as an enzyme inhibitor?
A: D-talono-1,4-lactone acts as a competitive inhibitor of β-galactosidases. [, , , ] This means it competes with the natural substrate for binding to the enzyme's active site. This interaction prevents the enzyme from breaking down its usual substrates, such as lactose, GM1-ganglioside, and lactosylceramide. [, , ]
Q2: How does the structure of D-talono-1,4-lactone contribute to its inhibitory activity?
A: The lactone ring structure of D-talono-1,4-lactone is similar to the transition state of the natural substrate during the hydrolysis reaction catalyzed by β-galactosidases. This structural similarity allows it to bind to the enzyme's active site with high affinity, leading to competitive inhibition. [, , , ]
Q3: Are there differences in the inhibitory potency of D-talono-1,4-lactone towards different β-galactosidase isoforms?
A: Yes, research indicates that the inhibitory potency of D-talono-1,4-lactone can vary depending on the specific β-galactosidase isoform. For instance, studies on a rabbit brain β-galactosidase showed different Ki values for the inhibition of GM1-ganglioside, lactosylceramide, and MU-galactoside hydrolysis. [] Similarly, different degrees of inhibition were observed for various substrates in the presence of chloromercuriphenylsulfonic acid, suggesting multiple active sites with varying affinities for the inhibitor. []
Disclaimer and Information on In-Vitro Research Products
Please be aware that all articles and product information presented on BenchChem are intended solely for informational purposes. The products available for purchase on BenchChem are specifically designed for in-vitro studies, which are conducted outside of living organisms. In-vitro studies, derived from the Latin term "in glass," involve experiments performed in controlled laboratory settings using cells or tissues. It is important to note that these products are not categorized as medicines or drugs, and they have not received approval from the FDA for the prevention, treatment, or cure of any medical condition, ailment, or disease. We must emphasize that any form of bodily introduction of these products into humans or animals is strictly prohibited by law. It is essential to adhere to these guidelines to ensure compliance with legal and ethical standards in research and experimentation.