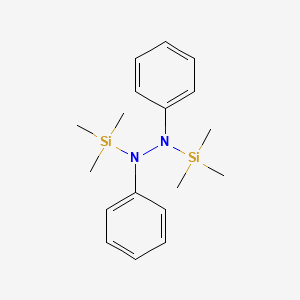
1,2-Bis(trimethylsilyl)-1,2-diphenylhydrazine
- Click on QUICK INQUIRY to receive a quote from our team of experts.
- With the quality product at a COMPETITIVE price, you can focus more on your research.
Overview
Description
1,2-Bis(trimethylsilyl)-1,2-diphenylhydrazine is an organosilicon compound that has garnered interest due to its unique structural properties and potential applications in various fields of chemistry. This compound features two trimethylsilyl groups and two phenyl groups attached to a hydrazine core, making it a versatile molecule for synthetic and functionalization studies.
Preparation Methods
Synthetic Routes and Reaction Conditions
1,2-Bis(trimethylsilyl)-1,2-diphenylhydrazine can be synthesized through several methods. One common approach involves the reaction of 1,2-diphenylhydrazine with trimethylsilyl chloride in the presence of a base such as triethylamine. The reaction typically occurs in an inert solvent like tetrahydrofuran (THF) under anhydrous conditions to prevent hydrolysis of the silyl groups.
Industrial Production Methods
While specific industrial production methods for this compound are not well-documented, the general principles of organosilicon chemistry can be applied. Large-scale synthesis would likely involve optimized reaction conditions to maximize yield and purity, including the use of high-purity reagents, controlled reaction temperatures, and efficient purification techniques such as distillation or recrystallization.
Chemical Reactions Analysis
Types of Reactions
1,2-Bis(trimethylsilyl)-1,2-diphenylhydrazine undergoes various chemical reactions, including:
Oxidation: The compound can be oxidized to form corresponding azo compounds.
Reduction: Reduction reactions can yield hydrazine derivatives.
Substitution: The trimethylsilyl groups can be substituted with other functional groups through nucleophilic substitution reactions.
Common Reagents and Conditions
Oxidation: Common oxidizing agents include hydrogen peroxide and potassium permanganate.
Reduction: Reducing agents such as lithium aluminum hydride (LiAlH4) or sodium borohydride (NaBH4) are typically used.
Substitution: Nucleophiles like Grignard reagents or organolithium compounds can facilitate substitution reactions.
Major Products
The major products formed from these reactions depend on the specific conditions and reagents used. For example, oxidation can yield azo compounds, while substitution reactions can introduce various functional groups, enhancing the compound’s versatility.
Scientific Research Applications
1,2-Bis(trimethylsilyl)-1,2-diphenylhydrazine has several applications in scientific research:
Chemistry: It serves as a precursor for the synthesis of other organosilicon compounds and can be used in the study of silicon-based π-electron systems.
Industry: The compound can be used in the production of advanced materials, including polymers and coatings, due to its unique structural properties.
Mechanism of Action
The mechanism of action of 1,2-Bis(trimethylsilyl)-1,2-diphenylhydrazine involves its ability to undergo various chemical transformations. The trimethylsilyl groups provide steric protection and can be selectively removed or substituted, allowing for the introduction of different functional groups. This versatility makes it a valuable intermediate in synthetic chemistry.
Comparison with Similar Compounds
Similar Compounds
1,2-Bis(trimethylsilyl)benzene: Similar in structure but lacks the hydrazine core.
1,2-Bis(trimethylsilyl)ethane: Another related compound with different reactivity due to the absence of phenyl groups.
1,2-Bis(trimethylsilyl)-1,2-disilacyclohexene: Features a silicon-silicon double bond, offering unique electronic properties.
Uniqueness
1,2-Bis(trimethylsilyl)-1,2-diphenylhydrazine is unique due to its combination of trimethylsilyl and phenyl groups attached to a hydrazine core. This structure provides a balance of steric protection and reactivity, making it a versatile compound for various synthetic applications.
Properties
CAS No. |
5994-95-6 |
---|---|
Molecular Formula |
C18H28N2Si2 |
Molecular Weight |
328.6 g/mol |
IUPAC Name |
1,2-diphenyl-1,2-bis(trimethylsilyl)hydrazine |
InChI |
InChI=1S/C18H28N2Si2/c1-21(2,3)19(17-13-9-7-10-14-17)20(22(4,5)6)18-15-11-8-12-16-18/h7-16H,1-6H3 |
InChI Key |
KZVGBFZABXRJFE-UHFFFAOYSA-N |
Canonical SMILES |
C[Si](C)(C)N(C1=CC=CC=C1)N(C2=CC=CC=C2)[Si](C)(C)C |
Origin of Product |
United States |
Disclaimer and Information on In-Vitro Research Products
Please be aware that all articles and product information presented on BenchChem are intended solely for informational purposes. The products available for purchase on BenchChem are specifically designed for in-vitro studies, which are conducted outside of living organisms. In-vitro studies, derived from the Latin term "in glass," involve experiments performed in controlled laboratory settings using cells or tissues. It is important to note that these products are not categorized as medicines or drugs, and they have not received approval from the FDA for the prevention, treatment, or cure of any medical condition, ailment, or disease. We must emphasize that any form of bodily introduction of these products into humans or animals is strictly prohibited by law. It is essential to adhere to these guidelines to ensure compliance with legal and ethical standards in research and experimentation.