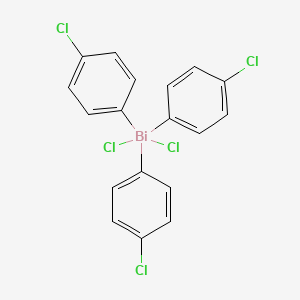
Bismuth, dichlorotris(4-chlorophenyl)-
- Click on QUICK INQUIRY to receive a quote from our team of experts.
- With the quality product at a COMPETITIVE price, you can focus more on your research.
Overview
Description
Bismuth, dichlorotris(4-chlorophenyl)-: is an organobismuth compound with the molecular formula C18H12BiCl5 . This compound is known for its unique structure, where a bismuth atom is coordinated with three 4-chlorophenyl groups and two chlorine atoms.
Preparation Methods
Synthetic Routes and Reaction Conditions: The synthesis of Bismuth, dichlorotris(4-chlorophenyl)- typically involves the reaction of bismuth trichloride with 4-chlorophenyl magnesium bromide in an inert atmosphere. The reaction is carried out in a solvent such as tetrahydrofuran at low temperatures to ensure the stability of the intermediate products. The general reaction can be represented as follows:
BiCl3+3C6H4ClMgBr→Bi(C6H4Cl)3Cl2+3MgBrCl
Industrial Production Methods: While the laboratory synthesis is well-documented, industrial production methods for this compound are less common due to its specialized applications. scaling up the laboratory methods with appropriate safety and environmental controls can be considered for industrial production .
Chemical Reactions Analysis
Types of Reactions:
Oxidation: Bismuth, dichlorotris(4-chlorophenyl)- can undergo oxidation reactions, where the bismuth center is oxidized to higher oxidation states.
Reduction: The compound can also be reduced under specific conditions, leading to the formation of lower oxidation state bismuth compounds.
Substitution: The chlorine atoms in the compound can be substituted by other ligands through nucleophilic substitution reactions.
Common Reagents and Conditions:
Oxidation: Common oxidizing agents include hydrogen peroxide and nitric acid.
Reduction: Reducing agents such as sodium borohydride or lithium aluminum hydride can be used.
Substitution: Nucleophiles like amines or phosphines can be employed for substitution reactions.
Major Products: The major products formed from these reactions depend on the specific reagents and conditions used. For example, oxidation may yield bismuth(V) compounds, while reduction could produce bismuth(I) species .
Scientific Research Applications
Chemistry: Bismuth, dichlorotris(4-chlorophenyl)- is used as a reagent in organic synthesis, particularly in the formation of carbon-bismuth bonds. It serves as a precursor for various organobismuth compounds.
Biology and Medicine: Research has explored the potential of organobismuth compounds in medicinal chemistry, particularly for their antimicrobial properties. Bismuth compounds are known to exhibit activity against a range of pathogens, including bacteria and fungi.
Industry: In the industrial sector, this compound can be used in the development of new materials with unique electronic and optical properties. Its high electrical resistance and Hall Effect make it suitable for specific electronic applications .
Mechanism of Action
The mechanism by which Bismuth, dichlorotris(4-chlorophenyl)- exerts its effects is primarily through its interaction with biological molecules. The bismuth center can coordinate with various biomolecules, disrupting their normal function. This coordination can inhibit the activity of enzymes or interfere with the structural integrity of cellular components. The exact molecular targets and pathways involved depend on the specific application and context of use .
Comparison with Similar Compounds
- Triphenylbismuth dichloride
- Tris(2-methoxyphenyl)bismuth dichloride
- Tris(4-trifluoromethylphenyl)bismuth dichloride
Comparison: Bismuth, dichlorotris(4-chlorophenyl)- is unique due to the presence of 4-chlorophenyl groups, which impart specific electronic and steric properties. Compared to other organobismuth compounds, it may exhibit different reactivity and stability profiles. For instance, the presence of electron-withdrawing chlorine atoms can influence the compound’s reactivity in substitution reactions .
Biological Activity
Bismuth, dichlorotris(4-chlorophenyl)- is an organometallic compound that has garnered attention for its potential biological activities, particularly in antimicrobial and anti-inflammatory applications. This article explores the biological activity of this compound, presenting data tables, case studies, and detailed research findings.
Chemical Structure and Properties
Bismuth, dichlorotris(4-chlorophenyl)- features a bismuth center coordinated with three 4-chlorophenyl groups and two chloride ions. The presence of chlorophenyl moieties enhances its reactivity and biological interactions. The compound's molecular formula is C12H8Cl2Bi, and its molecular weight is approximately 358.6 g/mol.
Biological Activity Overview
Bismuth compounds, including bismuth, dichlorotris(4-chlorophenyl)-, have demonstrated significant antimicrobial and anti-inflammatory properties. The following sections detail specific biological activities supported by research findings.
Antimicrobial Activity
Bismuth compounds are known for their antibacterial properties against a range of pathogens, including Helicobacter pylori (H. pylori) and methicillin-resistant Staphylococcus aureus (MRSA). The antimicrobial efficacy of bismuth is enhanced when combined with other agents.
Table 1: Antimicrobial Activity of Bismuth Compounds
Compound | Target Pathogen | MIC (µM) |
---|---|---|
Bismuth, dichlorotris(4-chlorophenyl)- | H. pylori | 2.2 |
Bismuth combined with thiols | MRSA | 5 - 7 |
Bismuth-thiol complex (BisBAL) | Various Gram-positive bacteria | <17 |
Research indicates that the antibacterial activity of bismuth can be significantly enhanced when combined with lipophilic thiol compounds. For instance, the combination of bismuth with dimercaprol (BAL) showed a 25- to 300-fold increase in antibacterial potency against various bacterial strains .
Anti-inflammatory Activity
Bismuth compounds have also been studied for their anti-inflammatory properties. In vitro studies suggest that these compounds can inhibit the production of pro-inflammatory cytokines, making them potential candidates for treating inflammatory diseases.
Case Study: Bismuth in Inflammatory Conditions
A study investigated the effects of bismuth-containing therapies on patients with inflammatory bowel disease (IBD). The results indicated that patients receiving bismuth-based treatments experienced a reduction in inflammatory markers compared to those on standard therapies .
The mechanisms underlying the biological activity of bismuth, dichlorotris(4-chlorophenyl)- are multifaceted:
- Interaction with Biological Molecules : Bismuth can bind to proteins and nucleic acids, altering their function and stability.
- Generation of Reactive Species : Bismuth compounds may induce oxidative stress in bacterial cells, leading to cell death.
- Synergistic Effects : The combination of bismuth with other agents (e.g., thiols) enhances its solubility and bioavailability, improving overall efficacy .
Pharmacokinetics
The pharmacokinetics of bismuth compounds are crucial for understanding their therapeutic potential. Studies have shown that bismuth is rapidly absorbed in the gastrointestinal tract, with a median time to peak concentration (tmax) of approximately 0.75 hours . The half-life varies between formulations but generally ranges from 11 to 13 hours.
Table 2: Pharmacokinetic Parameters of Bismuth
Parameter | Value |
---|---|
tmax | 0.75 hours |
Half-life | 11-13 hours |
Bioavailability | High |
Properties
CAS No. |
77760-10-2 |
---|---|
Molecular Formula |
C18H12BiCl5 |
Molecular Weight |
614.5 g/mol |
IUPAC Name |
dichloro-tris(4-chlorophenyl)bismuth |
InChI |
InChI=1S/3C6H4Cl.Bi.2ClH/c3*7-6-4-2-1-3-5-6;;;/h3*2-5H;;2*1H/q;;;+2;;/p-2 |
InChI Key |
XYIFZIMPBZNAJB-UHFFFAOYSA-L |
Canonical SMILES |
C1=CC(=CC=C1Cl)[Bi](C2=CC=C(C=C2)Cl)(C3=CC=C(C=C3)Cl)(Cl)Cl |
Origin of Product |
United States |
Disclaimer and Information on In-Vitro Research Products
Please be aware that all articles and product information presented on BenchChem are intended solely for informational purposes. The products available for purchase on BenchChem are specifically designed for in-vitro studies, which are conducted outside of living organisms. In-vitro studies, derived from the Latin term "in glass," involve experiments performed in controlled laboratory settings using cells or tissues. It is important to note that these products are not categorized as medicines or drugs, and they have not received approval from the FDA for the prevention, treatment, or cure of any medical condition, ailment, or disease. We must emphasize that any form of bodily introduction of these products into humans or animals is strictly prohibited by law. It is essential to adhere to these guidelines to ensure compliance with legal and ethical standards in research and experimentation.