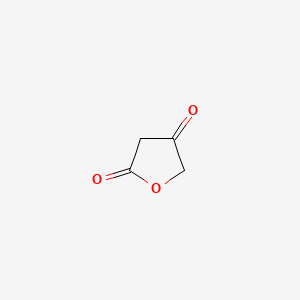
Tetronic acid
Overview
Description
It exists in equilibrium between keto and enol tautomers . This compound is notable for its presence in various natural products, including ascorbic acid (vitamin C), penicillic acid, pulvinic acids, and abyssomicins . Tetronic acid serves as a precursor in organic synthesis and forms the structural core of certain pesticides .
Mechanism of Action
Target of Action
Tetronic acid is a versatile synthon that has been extensively investigated due to its crucial role in synthesizing heterocycles . It is characterized by a low pKa and is likely to be deprotonated under physiological conditions . Its structure makes it an excellent chelator of metallic cations . It has been proposed that the this compound moiety could be a steric and electronic mimic for carboxylate, phosphate, or sulfate groups .
Mode of Action
This compound interconverts between keto and enol tautomers . This interconversion, along with its ability to chelate metallic cations, plays a significant role in its interaction with its targets . For instance, spirodiclofen, a this compound derivative, is known to inhibit de novo lipid biosynthesis .
Biochemical Pathways
The biosynthetic origin of this compound is from α-amino and α-hydroxy acids . The C2–C3 segment of the this compound core almost always stems from acetate, a homologous fatty acid, or from malonate while the O–C5–C4 string is provided by a suitable activated α-hydroxy carboxylate . In the case of chlorothricin, a this compound derivative, it acts as an inhibitor of pyruvate carboxylase that catalyzes the anaplerotic CO2 fixation on synthetic media and it is able to inhibit cholesterol biosynthesis via the mevalomalonate pathway .
Pharmacokinetics
Information on the ADME properties of this compound is currently limited. Given its chemical properties, it is expected to have good bioavailability due to its ability to form salts under physiological conditions .
Result of Action
The molecular and cellular effects of this compound’s action are diverse, largely due to the wide range of compounds that can be synthesized from it. For instance, many natural products such as ascorbic acid (vitamin C), penicillic acid, pulvinic acids, and abyssomicins possess the β-keto-γ-butyrolactone motif of this compound . These compounds have various biological activities, including antibiotic properties .
Action Environment
The action, efficacy, and stability of this compound and its derivatives can be influenced by environmental factors such as pH. Given its low pKa, this compound is likely to be deprotonated under physiological conditions, resulting in tetramate or tetronate salts . This property may affect its interaction with targets and its overall biological activity.
Biochemical Analysis
Biochemical Properties
Tetronic acid plays a significant role in biochemical reactions due to its ability to interact with various enzymes, proteins, and other biomolecules. It is known to chelate metal cations, making it an excellent binder of metallic ions such as sodium, calcium, and magnesium . This chelation property is crucial for its biological activity, as it can influence enzyme function and stability. This compound also interacts with enzymes involved in its biosynthesis and degradation, such as L-threonic acid dehydrogenase, which directs tetronic acids into different catabolic pathways .
Cellular Effects
This compound affects various types of cells and cellular processes. It has been shown to influence cell signaling pathways, gene expression, and cellular metabolism. For instance, this compound derivatives exhibit antibiotic, antiviral, antineoplastic, and anticoagulant activities . These effects are often mediated through the compound’s ability to chelate metal ions, which can alter the activity of metal-dependent enzymes and proteins . Additionally, this compound can impact cellular redox states and oxidative stress responses, further influencing cell function and viability .
Molecular Mechanism
The molecular mechanism of this compound involves its interaction with biomolecules at the molecular level. This compound can bind to metal ions, forming stable complexes that can inhibit or activate enzymes . This binding can lead to changes in enzyme conformation and activity, affecting various biochemical pathways. This compound also influences gene expression by modulating the activity of transcription factors and other regulatory proteins . These interactions can result in altered cellular responses and physiological effects.
Temporal Effects in Laboratory Settings
In laboratory settings, the effects of this compound can change over time due to its stability and degradation properties. This compound is relatively stable under standard laboratory conditions, but it can degrade over time, especially in the presence of strong oxidizing agents or alkalis . Long-term studies have shown that this compound can have sustained effects on cellular function, including prolonged changes in enzyme activity and gene expression . These temporal effects are important for understanding the compound’s potential therapeutic and toxicological properties.
Dosage Effects in Animal Models
The effects of this compound vary with different dosages in animal models. At low doses, this compound and its derivatives can exhibit beneficial effects, such as antimicrobial and anticancer activities . At high doses, this compound can cause toxic or adverse effects, including cellular toxicity and organ damage . These dosage-dependent effects highlight the importance of careful dose optimization in therapeutic applications.
Metabolic Pathways
This compound is involved in various metabolic pathways, including those related to its biosynthesis and degradation. It interacts with enzymes such as L-threonic acid dehydrogenase, which directs tetronic acids into specific catabolic pathways . These interactions can influence metabolic flux and the levels of metabolites in cells. This compound can also affect the metabolism of other compounds by modulating enzyme activity and cofactor availability .
Transport and Distribution
This compound is transported and distributed within cells and tissues through interactions with transporters and binding proteins. Its ability to chelate metal ions can facilitate its transport across cell membranes and its accumulation in specific cellular compartments . This compound can also bind to proteins that mediate its localization and distribution within cells, influencing its biological activity and effects .
Subcellular Localization
The subcellular localization of this compound is influenced by its chemical properties and interactions with biomolecules. This compound can be directed to specific cellular compartments through targeting signals and post-translational modifications . Its localization can affect its activity and function, as it can interact with enzymes and proteins in different cellular environments. Understanding the subcellular localization of this compound is important for elucidating its mechanism of action and potential therapeutic applications .
Preparation Methods
Synthetic Routes and Reaction Conditions: Tetronic acid can be synthesized through several methods:
Base-promoted Dieckmann cyclization: This method involves the cyclization of γ-hydroxylated or γ-halogenated β-ketoesters.
Lactonization of γ-acetoxy-β-keto esters: This process involves the formation of the lactone ring.
Debromination of α-bromothis compound: This method involves the removal of bromine atoms from α-bromothis compound.
One-pot synthesis: This method involves the tandem process of transesterification and subsequent Dieckmann cyclization from aryl- or heteroarylacetic acid esters and hydroxyacetic acid esters.
Industrial Production Methods: Industrial production of this compound typically involves large-scale synthesis using the aforementioned methods, optimized for yield and purity. The choice of method depends on the desired scale, cost, and specific application requirements.
Chemical Reactions Analysis
Tetronic acid undergoes various chemical reactions, including:
Oxidation: this compound can be oxidized to form various products, depending on the oxidizing agent used.
Reduction: Reduction reactions can convert this compound into different reduced forms.
Substitution: this compound can undergo substitution reactions, particularly involving the hydroxyl group.
Common Reagents and Conditions:
Oxidizing agents: Common oxidizing agents include potassium permanganate and hydrogen peroxide.
Reducing agents: Sodium borohydride and lithium aluminum hydride are commonly used reducing agents.
Substitution reactions: These reactions often involve nucleophiles such as amines and alcohols.
Major Products Formed:
Oxidation products: Various oxidized derivatives of this compound.
Reduction products: Reduced forms of this compound.
Substitution products: Substituted this compound derivatives.
Scientific Research Applications
Tetronic acid has a wide range of applications in scientific research:
Comparison with Similar Compounds
Tetronic acid is structurally and functionally similar to several other compounds:
Tetramic acid: Both tetronic and tetramic acids contain a γ-lactone ring, but tetramic acid has a pyrrolidine-2,4-dione structure.
Ascorbic acid (vitamin C): Ascorbic acid shares the β-keto-γ-butyrolactone motif with this compound.
Penicillic acid: This compound also possesses the β-keto-γ-butyrolactone motif.
Pulvinic acids: These acids share structural similarities with this compound.
Abyssomicins: These natural products contain the β-keto-γ-butyrolactone motif.
This compound’s uniqueness lies in its versatile reactivity and wide range of applications in various fields, from organic synthesis to medicinal chemistry.
Properties
IUPAC Name |
oxolane-2,4-dione | |
---|---|---|
Source | PubChem | |
URL | https://pubchem.ncbi.nlm.nih.gov | |
Description | Data deposited in or computed by PubChem | |
InChI |
InChI=1S/C4H4O3/c5-3-1-4(6)7-2-3/h1-2H2 | |
Source | PubChem | |
URL | https://pubchem.ncbi.nlm.nih.gov | |
Description | Data deposited in or computed by PubChem | |
InChI Key |
JCGNDDUYTRNOFT-UHFFFAOYSA-N | |
Source | PubChem | |
URL | https://pubchem.ncbi.nlm.nih.gov | |
Description | Data deposited in or computed by PubChem | |
Canonical SMILES |
C1C(=O)COC1=O | |
Source | PubChem | |
URL | https://pubchem.ncbi.nlm.nih.gov | |
Description | Data deposited in or computed by PubChem | |
Molecular Formula |
C4H4O3 | |
Source | PubChem | |
URL | https://pubchem.ncbi.nlm.nih.gov | |
Description | Data deposited in or computed by PubChem | |
DSSTOX Substance ID |
DTXSID30902467 | |
Record name | NoName_1711 | |
Source | EPA DSSTox | |
URL | https://comptox.epa.gov/dashboard/DTXSID30902467 | |
Description | DSSTox provides a high quality public chemistry resource for supporting improved predictive toxicology. | |
Molecular Weight |
100.07 g/mol | |
Source | PubChem | |
URL | https://pubchem.ncbi.nlm.nih.gov | |
Description | Data deposited in or computed by PubChem | |
CAS No. |
4971-56-6 | |
Record name | Tetronic acid | |
Source | CAS Common Chemistry | |
URL | https://commonchemistry.cas.org/detail?cas_rn=4971-56-6 | |
Description | CAS Common Chemistry is an open community resource for accessing chemical information. Nearly 500,000 chemical substances from CAS REGISTRY cover areas of community interest, including common and frequently regulated chemicals, and those relevant to high school and undergraduate chemistry classes. This chemical information, curated by our expert scientists, is provided in alignment with our mission as a division of the American Chemical Society. | |
Explanation | The data from CAS Common Chemistry is provided under a CC-BY-NC 4.0 license, unless otherwise stated. | |
Record name | Tetronic acid | |
Source | ChemIDplus | |
URL | https://pubchem.ncbi.nlm.nih.gov/substance/?source=chemidplus&sourceid=0004971566 | |
Description | ChemIDplus is a free, web search system that provides access to the structure and nomenclature authority files used for the identification of chemical substances cited in National Library of Medicine (NLM) databases, including the TOXNET system. | |
Record name | β-oxo-γ-butyrolactone | |
Source | European Chemicals Agency (ECHA) | |
URL | https://echa.europa.eu/substance-information/-/substanceinfo/100.023.289 | |
Description | The European Chemicals Agency (ECHA) is an agency of the European Union which is the driving force among regulatory authorities in implementing the EU's groundbreaking chemicals legislation for the benefit of human health and the environment as well as for innovation and competitiveness. | |
Explanation | Use of the information, documents and data from the ECHA website is subject to the terms and conditions of this Legal Notice, and subject to other binding limitations provided for under applicable law, the information, documents and data made available on the ECHA website may be reproduced, distributed and/or used, totally or in part, for non-commercial purposes provided that ECHA is acknowledged as the source: "Source: European Chemicals Agency, http://echa.europa.eu/". Such acknowledgement must be included in each copy of the material. ECHA permits and encourages organisations and individuals to create links to the ECHA website under the following cumulative conditions: Links can only be made to webpages that provide a link to the Legal Notice page. | |
Record name | TETRONIC ACID | |
Source | FDA Global Substance Registration System (GSRS) | |
URL | https://gsrs.ncats.nih.gov/ginas/app/beta/substances/N2B9NB89C0 | |
Description | The FDA Global Substance Registration System (GSRS) enables the efficient and accurate exchange of information on what substances are in regulated products. Instead of relying on names, which vary across regulatory domains, countries, and regions, the GSRS knowledge base makes it possible for substances to be defined by standardized, scientific descriptions. | |
Explanation | Unless otherwise noted, the contents of the FDA website (www.fda.gov), both text and graphics, are not copyrighted. They are in the public domain and may be republished, reprinted and otherwise used freely by anyone without the need to obtain permission from FDA. Credit to the U.S. Food and Drug Administration as the source is appreciated but not required. | |
Retrosynthesis Analysis
AI-Powered Synthesis Planning: Our tool employs the Template_relevance Pistachio, Template_relevance Bkms_metabolic, Template_relevance Pistachio_ringbreaker, Template_relevance Reaxys, Template_relevance Reaxys_biocatalysis model, leveraging a vast database of chemical reactions to predict feasible synthetic routes.
One-Step Synthesis Focus: Specifically designed for one-step synthesis, it provides concise and direct routes for your target compounds, streamlining the synthesis process.
Accurate Predictions: Utilizing the extensive PISTACHIO, BKMS_METABOLIC, PISTACHIO_RINGBREAKER, REAXYS, REAXYS_BIOCATALYSIS database, our tool offers high-accuracy predictions, reflecting the latest in chemical research and data.
Strategy Settings
Precursor scoring | Relevance Heuristic |
---|---|
Min. plausibility | 0.01 |
Model | Template_relevance |
Template Set | Pistachio/Bkms_metabolic/Pistachio_ringbreaker/Reaxys/Reaxys_biocatalysis |
Top-N result to add to graph | 6 |
Feasible Synthetic Routes
Q1: What is the basic structure of a tetronic acid?
A1: Tetronic acids are characterized by a 4-hydroxy-2(5H)-furanone ring. [] This structure can exist in two tautomeric forms, with the enol form being predominant.
Q2: What is the molecular formula and weight of this compound?
A2: The molecular formula for this compound is C4H4O3, and its molecular weight is 100.07 g/mol.
Q3: What are some key spectroscopic characteristics of tetronic acids?
A3: Spectroscopic techniques like NMR (Nuclear Magnetic Resonance) and IR (Infrared Spectroscopy) are crucial for identifying and characterizing tetronic acids. For instance, the 1H NMR spectra of tetronic acids typically display characteristic signals corresponding to the protons on the furanone ring. [, ] Additionally, the carbonyl groups in the this compound structure give rise to distinctive absorption bands in the IR spectrum. [, ]
Q4: How stable are tetronic acids under different conditions?
A4: While generally stable, the stability of tetronic acids can vary based on the substituents present and the environmental conditions. For example, certain spiroepoxy-beta-lactones, synthesized from ketene dimers, can rearrange into tetronic acids under various conditions, highlighting their relative stability. []
Q5: Are there any studies on the dissolution and solubility of tetronic acids?
A5: While the provided research doesn't delve deeply into the dissolution and solubility of all tetronic acids, understanding these properties is crucial for their potential use in pharmaceuticals. Factors like pH and the type of media can significantly influence solubility, which directly impacts bioavailability and efficacy. []
Q6: What are some common synthetic routes to access tetronic acids?
A6: Numerous synthetic strategies have been developed over the years, highlighting the versatility of these compounds:
- From α-(chloroacetyl)malonate: Treatment of readily prepared diethyl α-(chloroacetyl)malonate with triethylamine yields a key intermediate which can be converted to this compound and various substituted analogues. []
- From 1,3-dioxolan-4-ones: This approach offers a versatile route to both 2-substituted and 3-substituted this compound derivatives by reacting the dioxolanone with appropriate nucleophiles. []
- From 1,3-dioxin-4-ones: This method exploits the cycloreversion of 6-substituted 1,3-dioxin-4-ones to acylketenes, which can then be trapped intramolecularly or intermolecularly to furnish diverse this compound derivatives. []
- From (S)-ketone cyanohydrins: This enantioselective approach utilizes biocatalysts to access enantiomerically pure starting materials, ultimately leading to optically active 5,5-disubstituted tetronic acids with high enantiomeric excesses. []
- Solid-phase synthesis: This approach offers a convenient and efficient method for the synthesis of functionalized tetronic acids, utilizing a carbonate linker for ease of manipulation and mild cleavage conditions. []
Q7: How can tetronic acids be alkylated?
A7: The 4-oxygen of tetronic acids can be selectively alkylated, a useful transformation for synthesizing various derivatives:
- Acid-catalyzed etherification: Treatment of tetronic acids with HCl in different alcohols yields the corresponding 4-alkoxy-5H-furan-2-ones. []
- Using oxyphosphonium intermediates: This highly efficient method involves treating tetronic acids with triphenylphosphonium anhydride trifluoromethanesulfonate, facilitating regioselective alkylation with both primary and secondary alcohols. []
Q8: Can tetronic acids participate in cycloaddition reactions?
A8: Yes, studies have shown that this compound derivatives with strategically placed alkenes can undergo [2+2] photocycloaddition reactions. These reactions demonstrate potential for building complex structures, as exemplified by the synthesis of cembranoid diterpenes. []
Q9: What are some prominent biological activities exhibited by tetronic acids?
A9: Tetronic acids and their derivatives display a wide array of biological activities, making them attractive targets for drug discovery:
- Hypocholesterolemic and antiaggregatory properties: Certain derivatives, like 4-(4-chlorophenyl)-2-hydroxythis compound, have demonstrated potential in lowering cholesterol levels and inhibiting platelet aggregation. []
- Protein phosphatase inhibition: 3-Acylthis compound derivatives have shown promise as inhibitors of protein tyrosine phosphatases (PTPs) and dual-specificity phosphatases (DSPs), particularly cdc25B, a key enzyme in cell cycle regulation. []
- Antimicrobial activity: Compounds like pestalotic acids A-G, featuring a furylidene this compound core, exhibit significant antimicrobial properties. []
- HIV-1 protease inhibition: 3-Alkanoyl-5-hydroxymethyl this compound homologues and resistomycin have shown inhibitory activity against HIV-1 protease. []
- Fungicidal and insecticidal activity: Various this compound hydrazinyl derivatives demonstrate potent fungicidal and insecticidal activity against a range of plant pathogens and pests. []
Q10: How do tetronic acids interact with their biological targets?
A10: The mechanism of action varies depending on the specific this compound derivative and its target. For instance, in the case of protein phosphatase inhibition, 3-acyltetronic acids likely act as phosphate mimics, competing for the active site of the enzyme. [] Further research is needed to fully elucidate the specific interactions and downstream effects for various this compound derivatives.
Q11: Are there any known cases of resistance to this compound-based compounds?
A11: While the provided research doesn't specifically address resistance mechanisms, it is a crucial aspect to consider when developing new drugs. Understanding potential resistance mechanisms and exploring strategies to overcome them will be crucial for the long-term success of this compound-based therapies.
Q12: How has computational chemistry been employed in this compound research?
A12: Computational tools are increasingly being used to understand and predict the properties and activities of tetronic acids:
- QSAR (Quantitative Structure-Activity Relationship) studies: By correlating chemical structure with biological activity, QSAR models can help guide the design of more potent and selective this compound derivatives. Studies have successfully used 1H NMR and 13C NMR chemical shifts to develop QSAR models for the antifungal activity of phenylhydrazine-substituted tetronic acids. []
Q13: How do modifications to the this compound structure influence activity?
A13: The structure-activity relationship (SAR) is a critical aspect of drug development.
- Substitution at the 3-position: This position offers significant opportunities for modulating biological activity. For example, 3-carboethoxy derivatives stimulate rice root growth, while removing the carboethoxy group leads to growth inhibition. []
- Substitution at the 5-position: This position also plays a crucial role in determining biological activity. For example, incorporating a spiro cyclohexyl moiety at the 5-position significantly enhances the fungicidal activity of this compound derivatives. []
- Introduction of various functional groups: Introducing different functional groups, such as hydrazinyl groups, can significantly alter biological activity, as seen with the increased insecticidal and acaricidal activities observed in certain derivatives. []
Disclaimer and Information on In-Vitro Research Products
Please be aware that all articles and product information presented on BenchChem are intended solely for informational purposes. The products available for purchase on BenchChem are specifically designed for in-vitro studies, which are conducted outside of living organisms. In-vitro studies, derived from the Latin term "in glass," involve experiments performed in controlled laboratory settings using cells or tissues. It is important to note that these products are not categorized as medicines or drugs, and they have not received approval from the FDA for the prevention, treatment, or cure of any medical condition, ailment, or disease. We must emphasize that any form of bodily introduction of these products into humans or animals is strictly prohibited by law. It is essential to adhere to these guidelines to ensure compliance with legal and ethical standards in research and experimentation.